Registration Dossier
Registration Dossier
Data platform availability banner - registered substances factsheets
Please be aware that this old REACH registration data factsheet is no longer maintained; it remains frozen as of 19th May 2023.
The new ECHA CHEM database has been released by ECHA, and it now contains all REACH registration data. There are more details on the transition of ECHA's published data to ECHA CHEM here.
Diss Factsheets
Use of this information is subject to copyright laws and may require the permission of the owner of the information, as described in the ECHA Legal Notice.
EC number: 828-229-9 | CAS number: 7019-19-4
- Life Cycle description
- Uses advised against
- Endpoint summary
- Appearance / physical state / colour
- Melting point / freezing point
- Boiling point
- Density
- Particle size distribution (Granulometry)
- Vapour pressure
- Partition coefficient
- Water solubility
- Solubility in organic solvents / fat solubility
- Surface tension
- Flash point
- Auto flammability
- Flammability
- Explosiveness
- Oxidising properties
- Oxidation reduction potential
- Stability in organic solvents and identity of relevant degradation products
- Storage stability and reactivity towards container material
- Stability: thermal, sunlight, metals
- pH
- Dissociation constant
- Viscosity
- Additional physico-chemical information
- Additional physico-chemical properties of nanomaterials
- Nanomaterial agglomeration / aggregation
- Nanomaterial crystalline phase
- Nanomaterial crystallite and grain size
- Nanomaterial aspect ratio / shape
- Nanomaterial specific surface area
- Nanomaterial Zeta potential
- Nanomaterial surface chemistry
- Nanomaterial dustiness
- Nanomaterial porosity
- Nanomaterial pour density
- Nanomaterial photocatalytic activity
- Nanomaterial radical formation potential
- Nanomaterial catalytic activity
- Endpoint summary
- Stability
- Biodegradation
- Bioaccumulation
- Transport and distribution
- Environmental data
- Additional information on environmental fate and behaviour
- Ecotoxicological Summary
- Aquatic toxicity
- Endpoint summary
- Short-term toxicity to fish
- Long-term toxicity to fish
- Short-term toxicity to aquatic invertebrates
- Long-term toxicity to aquatic invertebrates
- Toxicity to aquatic algae and cyanobacteria
- Toxicity to aquatic plants other than algae
- Toxicity to microorganisms
- Endocrine disrupter testing in aquatic vertebrates – in vivo
- Toxicity to other aquatic organisms
- Sediment toxicity
- Terrestrial toxicity
- Biological effects monitoring
- Biotransformation and kinetics
- Additional ecotoxological information
- Toxicological Summary
- Toxicokinetics, metabolism and distribution
- Acute Toxicity
- Irritation / corrosion
- Sensitisation
- Repeated dose toxicity
- Genetic toxicity
- Carcinogenicity
- Toxicity to reproduction
- Specific investigations
- Exposure related observations in humans
- Toxic effects on livestock and pets
- Additional toxicological data
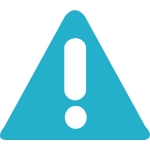
Endpoint summary
Administrative data
Link to relevant study record(s)
- Endpoint:
- basic toxicokinetics, other
- Type of information:
- other: Assessment of toxicokinetic behaviour based on literature data, knowledge of functional group metabolism pathways and phys-chem properties of 1-hydroxyoctan-2-one
- Adequacy of study:
- key study
- Study period:
- 2018
- Reliability:
- 4 (not assignable)
- Rationale for reliability incl. deficiencies:
- secondary literature
- GLP compliance:
- no
- Metabolites identified:
- yes
- Details on metabolites:
- Existing literature data concerning biotransformation pathways for aliphatic ketones and hydroxyketones (EFSA, 2014; WHO 2011a; WHO 2011b), show the potential metabolic reactions involved in the biotransformation of 1-hydroxyoctan-2-one are:
(i) Reduction of the ketone functional group to yield the corresponding diol (caprylyl glycol) with subsequent excretion in urine or bile as conjugate of glucuronic acid;
(ii) Oxidation of the terminal hydroxyl group to yield α-ketocarboxylic acid (an intermediary metabolite) which may undergo oxidative decarboxylation to yield carbon dioxide and an aliphatic carboxylic acid which may be completely metabolised via fatty acids and the krebs cycle. - Conclusions:
- In general, aliphatic secondary alcohols and ketones (functional groups present in the registered substance 1-hydroxyoctan-2-one) are expected to be rapidly absorbed in the gastrointestinal tract (EFSA, 2014). Specifically, significant absorption and systemic availability of 1-hydroxyoctan-2-one or metabolites after oral administration can be concluded from its moderately high water solubility (9.2 g/L at 20°C), low octanol-water partition coefficient (Log Kow = 1.68) and its relatively low molecular weight (144.2 g/mol). These three physicochemical properties also favour dermal uptake, although the systemic availability of 1-hydroxyoctan-2-one after dermal uptake is considered to be lower than after gastro-intestinal absorption following exposure via the oral route.
- Executive summary:
No specific experimental study data is available on the absorption, distribution, metabolism and/or excretion (ADME) profile of 1-hydroxyoctan-2-one. However, the toxicokinetic behaviour of the substancein vivocan be estimated using physic-chemical properties of the substance combined with data on structurally analogous compounds.
In general, aliphatic secondary alcohols and ketones are expected to be rapidly absorbed in the gastrointestinal tract (EFSA, 2014). Specifically, significant absorption and systemic availability of 1-hydroxyoctan-2-one or metabolites after oral administration can be concluded from its moderately high water solubility (9.2 g/L at 20°C), low octanol-water partition coefficient (Log Kow = 1.68) and its relatively low molecular weight (144.2 g/mol). These three physicochemical properties also favour dermal uptake, although the systemic availability of 1-hydroxyoctan-2-one after dermal uptake is considered to be lower than after gastro-intestinal absorption following exposure via the oral route.
Although the low octanol-water partition coefficient (log Kow) and the moderately high water solubility, indicate absorption directly across the respiratory tract epithelium by passive diffusion, the risk of exposure by the inhalation route for 1-hydroxyoctan-2-one is considered to be low, because of its relatively low vapour pressure (27 Pa at 20°C).
Existing literature data concerning biotransformation pathways for aliphatic ketones and hydroxyketones (EFSA, 2014; WHO 2011a; WHO 2011b), show the potential metabolic reactions involved in the biotransformation of 1-hydroxyoctan-2-one are:
(i) Reduction of the ketone functional group to yield the corresponding diol (caprylyl glycol) with subsequent excretion in urine or bile as conjugate of glucuronic acid;
(ii) Oxidation of the terminal hydroxyl group to yield α-ketocarboxylic acid (an intermediary metabolite) which may undergo oxidative decarboxylation to yield carbon dioxide and an aliphatic carboxylic acid which may be completely metabolised via fatty acids and the krebs cycle.
According to the available literature, reduction of the ketone group in aliphatic α-hydroxyketones (i.e. metabolic transformation route (i) above) is favoured at elevatedin vivoconcentrations, especially for longer chain length ketones (EFSA, 2014).
An overview of the possible biotransformation pathways involved in the metabolism of 1-hydroxyoctan-2-one is provided in the attached Figure 1.
It is noted that α-hydroxy ketones (i.e.1-hydroxyoctan-2-one)exist in equilibrium with α-hydroxy aldehydes (i.e. 2-hydroxyoctanal) via enediol keto-enol tautomerism. However the inductive effect caused by the adjacent hydroxyl group intensifies electrophilicity of the α-carbon and increases the rate of enolization and conversion of aldehyde into the more stable keto form which predominates (Vaismaa, 2009). The α-hydroxyl also makes the aldehyde carbon highly reactive, which have been exploited in natural processes. Due to this high reactivity it can be reasonably anticipated that even if the aldehyde form of 1-hydroxyoctan-2-one (i.e. 2-hydroxyoctanal) were to existin vivo, it would be expected (but not proven experimentally) to be subject to oxidation and conversion toα-ketocarboxylic acid (via α-hydroxycarboxylicacid) as shown in the attached Figure 1.
In view of its high water solubility, a Log octanol-water partition coefficient (Log Kow) value of 1.68 and anticipated susceptibility to undergo rapid metabolism and elimination processesin vivo, the potential for bioaccumulation of 1-hydroxyoctan-2-one is not considered to be of concern.
References:
EFSA ANS Panel (EFSA Panel on Food Additives and Nutrient Sources added to Food), Mortensen A, Aguilar F, Crebelli R, Di Domenico A, Dusemund B, Frutos MJ, Galtier P, Gott D, Gundert‐Remy U, Leblanc J‐C, Lindtner O, Moldeus P, Mosesso P, Parent‐Massin D, Oskarsson A, Stankovic I, Waalkens‐Berendsen I, Woutersen RA, Wright M, Younes M, Boon P, Chrysafidis D, Gürtler R, Tobback P, Gergelova P, Rincon AM and Lambré C, 2017. Scientific Opinion on the re‐evaluation of fatty acids (E 570) as a food additive. EFSA Journal 2017;15(5):4785, 48 pp.https://doi.org/10.2903/j.efsa.2017.4785
EFSA CEF Panel (EFSA Panel on Food Contact Materials, Enzymes, Flavourings and Processing Aids), 2014. Scientific Opinion on Flavouring Group Evaluation 11, Revision 3 (FGE.11Rev3): Aliphatic dialcohols, diketones, and hydroxyketones from chemical groups 8 and 10. EFSA Journal 2014;12(11):3888, 60 pp.http://www.efsa.europa.eu/en/efsajournal/pub/3888
Vaismaa M. (2009). Development of Benign Synthesis of Some Terminal α-Hydroxy Ketones and Aldehydes. Faculty of Science, Department of Chemistry, University of Oulu, P.O. Box 3000, FI-90014 University of Oulu, Finland Acta Univ. Oul. A 532, 2009.http://jultika.oulu.fi/files/isbn9789514291753.pdf
WHO (2011a). WHO Food Additives Series: 64. Safety evaluation of certain food additives and contaminants. Prepared by the Seventy-third meeting of the Joint FAO/WHO Expert Committee on Food Additives (JECFA).
http://apps.who.int/iris/bitstream/handle/10665/44521/9789241660648_eng.pdf?sequence=1&ua=1
WHO (2011b). Evaluation of certain food additives and contaminants. Seventy-third report of the Joint FAO/WHO Expert Committee on Food Additives. Geneva, 8–17 June 2010. WHO Technical Report Series, No 960. WHO, Geneva, Switzerland.
http://apps.who.int/iris/bitstream/handle/10665/44515/WHO_TRS_960_eng.pdf
Reference
Description of key information
No specific experimental study data is available on the absorption, distribution, metabolism and/or excretion (ADME) profile of 1-hydroxyoctan-2-one. However, the toxicokinetic behaviour of the substancein vivocan be estimated using physic-chemical properties of the substance combined with data on structurally analogous compounds.
In general, aliphatic secondary alcohols and ketones are expected to be rapidly absorbed in the gastrointestinal tract (EFSA, 2014). Specifically, significant absorption and systemic availability of 1-hydroxyoctan-2-one or metabolites after oral administration can be concluded from its moderately high water solubility (9.2 g/L at 20°C), low octanol-water partition coefficient (Log Kow = 1.68) and its relatively low molecular weight (144.2 g/mol). These three physicochemical properties also favour dermal uptake, although the systemic availability of 1-hydroxyoctan-2-one after dermal uptake is considered to be lower than after gastro-intestinal absorption following exposure via the oral route.
Although the low octanol-water partition coefficient (log Kow) and the moderately high water solubility, indicate absorption directly across the respiratory tract epithelium by passive diffusion, the risk of exposure by the inhalation route for 1-hydroxyoctan-2-one is considered to be low, because of its relatively low vapour pressure (27 Pa at 20°C).
Existing literature data concerning biotransformation pathways for aliphatic ketones and hydroxyketones (EFSA, 2014; WHO 2011a; WHO 2011b), show the potential metabolic reactions involved in the biotransformation of 1-hydroxyoctan-2-one are:
(i) Reduction of the ketone functional group to yield the corresponding diol (caprylyl glycol) with subsequent excretion in urine or bile as conjugate of glucuronic acid;
(ii) Oxidation of the terminal hydroxyl group to yield α-ketocarboxylic acid (an intermediary metabolite) which may undergo oxidative decarboxylation to yield carbon dioxide and an aliphatic carboxylic acid which may be completely metabolised via fatty acids and the krebs cycle.
According to the available literature, reduction of the ketone group in aliphatic α-hydroxyketones (i.e. metabolic transformation route (i) above) is favoured at elevatedin vivoconcentrations, especially for longer chain length ketones (EFSA, 2014).
An overview of the possible biotransformation pathways involved in the metabolism of 1-hydroxyoctan-2-one is provided in the attached Figure 1.
It is noted that α-hydroxy ketones (i.e.1-hydroxyoctan-2-one)exist in equilibrium with α-hydroxy aldehydes (i.e. 2-hydroxyoctanal) via enediol keto-enol tautomerism. However the inductive effect caused by the adjacent hydroxyl group intensifies electrophilicity of the α-carbon and increases the rate of enolization and conversion of aldehyde into the more stable keto form which predominates (Vaismaa, 2009). The α-hydroxyl also makes the aldehyde carbon highly reactive, which have been exploited in natural processes. Due to this high reactivity it can be reasonably anticipated that even if the aldehyde form of 1-hydroxyoctan-2-one (i.e. 2-hydroxyoctanal) were to existin vivo, it would be expected (but not proven experimentally) to be subject to oxidation and conversion toα-ketocarboxylic acid (via α-hydroxycarboxylicacid) as shown in the attached Figure 1.
In view of its high water solubility, a Log octanol-water partition coefficient (Log Kow) value of 1.68 and anticipated susceptibility to undergo rapid metabolism and elimination processesin vivo, the potential for bioaccumulation of 1-hydroxyoctan-2-one is not considered to be of concern.
Key value for chemical safety assessment
- Bioaccumulation potential:
- low bioaccumulation potential
Additional information
Information on Registered Substances comes from registration dossiers which have been assigned a registration number. The assignment of a registration number does however not guarantee that the information in the dossier is correct or that the dossier is compliant with Regulation (EC) No 1907/2006 (the REACH Regulation). This information has not been reviewed or verified by the Agency or any other authority. The content is subject to change without prior notice.
Reproduction or further distribution of this information may be subject to copyright protection. Use of the information without obtaining the permission from the owner(s) of the respective information might violate the rights of the owner.
