Registration Dossier
Registration Dossier
Data platform availability banner - registered substances factsheets
Please be aware that this old REACH registration data factsheet is no longer maintained; it remains frozen as of 19th May 2023.
The new ECHA CHEM database has been released by ECHA, and it now contains all REACH registration data. There are more details on the transition of ECHA's published data to ECHA CHEM here.
Diss Factsheets
Use of this information is subject to copyright laws and may require the permission of the owner of the information, as described in the ECHA Legal Notice.
EC number: 215-237-7 | CAS number: 1314-60-9
- Life Cycle description
- Uses advised against
- Endpoint summary
- Appearance / physical state / colour
- Melting point / freezing point
- Boiling point
- Density
- Particle size distribution (Granulometry)
- Vapour pressure
- Partition coefficient
- Water solubility
- Solubility in organic solvents / fat solubility
- Surface tension
- Flash point
- Auto flammability
- Flammability
- Explosiveness
- Oxidising properties
- Oxidation reduction potential
- Stability in organic solvents and identity of relevant degradation products
- Storage stability and reactivity towards container material
- Stability: thermal, sunlight, metals
- pH
- Dissociation constant
- Viscosity
- Additional physico-chemical information
- Additional physico-chemical properties of nanomaterials
- Nanomaterial agglomeration / aggregation
- Nanomaterial crystalline phase
- Nanomaterial crystallite and grain size
- Nanomaterial aspect ratio / shape
- Nanomaterial specific surface area
- Nanomaterial Zeta potential
- Nanomaterial surface chemistry
- Nanomaterial dustiness
- Nanomaterial porosity
- Nanomaterial pour density
- Nanomaterial photocatalytic activity
- Nanomaterial radical formation potential
- Nanomaterial catalytic activity
- Endpoint summary
- Stability
- Biodegradation
- Bioaccumulation
- Transport and distribution
- Environmental data
- Additional information on environmental fate and behaviour
- Ecotoxicological Summary
- Aquatic toxicity
- Endpoint summary
- Short-term toxicity to fish
- Long-term toxicity to fish
- Short-term toxicity to aquatic invertebrates
- Long-term toxicity to aquatic invertebrates
- Toxicity to aquatic algae and cyanobacteria
- Toxicity to aquatic plants other than algae
- Toxicity to microorganisms
- Endocrine disrupter testing in aquatic vertebrates – in vivo
- Toxicity to other aquatic organisms
- Sediment toxicity
- Terrestrial toxicity
- Biological effects monitoring
- Biotransformation and kinetics
- Additional ecotoxological information
- Toxicological Summary
- Toxicokinetics, metabolism and distribution
- Acute Toxicity
- Irritation / corrosion
- Sensitisation
- Repeated dose toxicity
- Genetic toxicity
- Carcinogenicity
- Toxicity to reproduction
- Specific investigations
- Exposure related observations in humans
- Toxic effects on livestock and pets
- Additional toxicological data
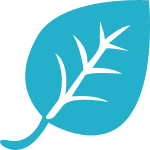
Endpoint summary
Administrative data
Description of key information
- especially for Sb(V), reliable data for many equilibria of interest in biological and environmental systems are lacking;
- a majority of the equilibration data have been generated at pH <5, i.e., not covering the pH domain of interest in environmental and biological systems;
- knowledge on the complex biotic and abiotic parameters/processes that govern the oxidation and reduction of antimony is limited;
- data for a reliable integration of complexants such as iron and manganese oxides, aluminosilicates and natural organic matter in the thermodynamic calculations are insufficient/lacking.
-
The solubility of Sb compounds in different environmental compartments depends on pH/Eh conditions but also on the time to dissolve; redox conditions determine their valence state. The results of transformation/dissolution tests indicate that some antimony substances may be more soluble at higher pH (e. g. pH 8.5 for Sb(V) substances) than at low pH (e. g. pH 6, for Sb(III) substances) (Skeaff et al., 2012)
-
In natural waters antimony exists almost exclusively in the dissolved phase in both the trivalent and pentavalent state. Both Sb(III) and Sb(V) ions hydrolyse easily: soluble Sb(III) is present as the neutral species Sb(OH)3, whereas soluble Sb(V) occurs as an anion, i.e. Sb(OH)6-. Both species may interact in natural waters with predominantly negatively charged natural organic matter, but firm conclusions cannot currently be drawn on its overall importance in Sb speciation (RAR).
-
In spite of thermodynamic predictions, who indicate that antimony should almost exclusively be present as Sb(V) in oxic systems and as Sb(III) in anoxic systems, concentrations of thermodynamically unstable antimony species have been measured in oxic and in anoxic systems. Antimony oxidation processes in laboratory solutions are slow, with half-lives usually being in the order of months when O2is the sole oxidant. The presence of factors such as UV light, dissolved organic carbon (DOC), and iron and manganese oxyhydroxide precipitates can all increase the rate of oxidation of Sb(III) to Sb(V), but available speciation monitoring data do demonstrate that thermodynamic equilibrium is unlikely to be approached in natural waters.
-
In terms of percentage of compound dissolved as calculated from the concentration of total dissolved Sb from the 1 mg/L loadings at 28 days, the reactivity/solubility of Sb metal in the T/Dp test was 57% and 61% at pH 6 and pH8.5, respectively.
-
The observed decrease in total dissolved Sb and in Sb(III) during the 28d T/Dp tests with Sb metal could be due to the formation of insoluble hydrolyzed antimonites.
-
In soil (and sediments), the most important property to influence the mobility of antimony appears to be the presence of hydrous oxides of Fe, Mn and Al, to which antimony may adsorb. In addition, these hydrous oxides seem to oxidise dissolved trivalent antimonite (Sb(OH)3) to the pentavalent antimonate (Sb(OH)6-).
- Due to its anionic character, the dissolved fraction of this pentavalent phase is not expected to interact significantly with the clay fraction in soil and sediment (i.e. cationic exchange reactions). Similarly, (Sb(OH)6-) is expected to have a low affinity for organic carbon in soil (and water). However, there are data that indicate that the sorption of pentavalent Sb by humic acid and/or organic matter may be more important than previously suspected. The strong Sb(V) scavenging potential of Fe(OH)3 probably results in a diminished role of organic matter as binding matrix; This would be, for instance, the case in soils with high amounts of amorphous hydroxides probably results in a diminished role of organic matter as binding matrix; This would be, for instance, the case in soils with high amounts of amorphous hydroxides.
-
The largest effect of pH on sorption seems to be around pH 3 - 4, with decreasing sorption at higher pH values. The effect of pH as such is probably less important when compared to the effect of the hydrous oxides.
-
In addition to the inorganic forms of antimony, there also exist methylated forms of trivalent and pentavalent antimony.
Due to a variety dynamic processes between different antimony speciation phases/valence states that are governed by environmental conditions, a read-across approach among simple antimony compounds is followed for the environmental fate evaluation. Dissolved antimony is the starting point of this approach.
Antimony metal and antimony containing compounds will dissolve and generate antimony ions (Vangheluwe et al.,2001). As antimony is an inorganic element, it cannot degrade. It may, however, be transformed between different phases, chemical species, and oxidation states.
The speciation and physico-chemical state of antimony are important to predict and understand its behaviour in the environment and availability to biota. Most analytical methods for antimony, however, do not distinguish between the various forms of antimony. While the total amount of antimony may be known, the nature of the antimony compounds, the importance of adsorption, etc. are not. This information, which is critical in determining the availability of antimony, is likely to be site-specific (ATSDR, 1992). Skeaff et al. (2012) examined the solubility and speciation of dissolved antimony in 7/28d transformation/dissolution tests with nine different antimony compounds: sodium hexahydroxoantimonate (NaSb(OH)6), antimony metal (Sb), antimony trioxide (Sb2O3), antimony sulfide (Sb2S3), sodium antimonate (NaSbO3), antimony tris(ethylene glycolate) (Sb2(C2H4O2)3), antimony trichloride (SbCl3), antimony triacetate (Sb(CH3COO)3), and antimony pentoxide (Sb2O5). Dissolved antimony form pentavalent Sb-compounds remained in the pentavalent valence state after 28 days, whereas the majority of Sb released from the other compounds remained in the trivalent state; some oxidation to the pentavalent state was noted for Sb released from Sb-metal, SbCl3 and Sb2S3. The kinetic stability of Sb(OH)3 that was formed during dissolution of Sb2O3, or the presence of the counter-ligand that was released upon dissolution (C2H4O22 - or CH3COO-) apparently stabilized the trivalent Sb in solution for the three remaining compounds. It should be noted that the T/D test medium is expected – apart form dissolved oxygen – to contain no oxidizing substances that normally occur under natural environmental conditions.
Valence of Sb in the environment
According to thermodynamic predictions – as generally accepted and repeated in many articles - antimony should almost exclusively be present as Sb(V) in oxic systems and as Sb(III) in anoxic systems, when they reach equilibrium. The use of the limited number of existing thermodynamic data for both valence states, does allow to consider this prediction reliable (Filella et al., 2009). Nevertheless, even though the dominant species in oxic waters is Sb(V), Sb(III) has been detected in concentrations much above what is predicted, and the reverse is true for Sb(V) in anoxic systems (EU RAR); such findings seem to be in accordance with the observations from Skeaff et al. (2012) who still found significant levels of trivalent Sb in oxic solutions after 28 days. An extremely slow oxidation rate of Sb(III) in aqueous solution when O2 was the sole oxidant in solution was indicated by Leuz and Johnson (2005): no significant oxidation of Sb(III) within 200 days was noted in homogenous aqueous solutions under environmental conditions (pH range of 3.6–9.8). Under normal environmental conditions, on the other hand, Sb(V) was by far the predominant species present in oxic systems with a low amount of Sb(III) sometimes present but rarely representing more than 10% of total antimony in the environment. This was clearly demonstrated by Filella et al. (2002a) who provided a comprehensive revision of published data on Sb-speciation in natural freshwaters, seawater and estuaries.
These contradicting observations for laboratory and natural environmental conditions are due to the fact that many of the processes that enhance the oxidation rate of trivalent antimony in the field do not occur in articicial laboratory media. Factors that increase the oxidation to Sb(V) are the presence of iron and manganese (hydr)oxides (Bilzele et al, 2001; Leuz et al., 2006), hydrogen peroxide (Quentel et al. 2004), and also the presence of humic acids (Buschmann and Sigg, 2004; Ceriotti and Amarasiriwardena, 2009). These substances are not present in e.g. reconstituted laboratory waters that are commonly used in speciation or (T/Dp) solubility experiments. For example, Leuz et al. (2006) reported a co-oxidation reaction pathway of Sb(III) with Fe2 + by both O2and H2O2and also provided evidence that there are increasing oxidation rate coefficients of Sb(III) in the presence of Fe2 + and O2with increasing pH. At neutral pH values, 45% of initial Sb(III) was oxidized at a Fe:Sb ratio of 1:1 within 60 min.
The residual fraction of observed Sb(III) and Sb(V) under oxic/anoxic conditions, respectively, could be attributed to the (continuous) introduction of ‘new’ Sb: a possible external source of Sb(III) in oxic surface waters is atmospheric deposition, whereas possible sources of Sb(V) in anoxic media are the transport of Sb(V) on sinking detritus from oxic waters or advection of oxic waters containing high Sb(V) concentrations (Filella et al., 2009). Conclusive evidence for such contributions, however, has not been established yet at this point in time.
Speciation of Sb in the environment
Antimony will not only be present in different valence states, but also in different speciation phases for each valence state. There are several approaches at hand for determining Sb-speciation in the environment: estimation through Eh-pH diagrams and/or speciation modeling, or conducting field measurements.
The relevance and reliability of both Eh-pH diagrams and using speciation models that cover different environmental compartments rely on the quality of the underlying thermodynamic data. There are indeed uncertainties surrounding the thermodynamic data for Sb compounds and, as a consequence, the Eh-pH diagrams differ between different sources. Earlier diagrams suggest that antimony is immobile under oxidizing conditions, occurring as solid oxides (Brookins, 1988), but more recent diagrams show that in oxidizing conditions, Sb(OH)6 - is the most important species, confirming the relatively high mobility of Sb under oxidizing conditions (Filella et al., 2002a; Filella et al., 2002b). In an effort to explain phase solubility relationships in metallurgical and geochemical processes, some of the reported diagrams do not only involve oxide phases and aqueous or hydrolysed species for both valence states, but also include the minerals stibnite (Sb2S3) and kermesite (Sb2S2O) for given activities of sulfate ion.
The reliability of some diagrams, however, remains questionable because inaccurate thermochemical parameters were available at the time of their construction, or because of the arbitrary selection of thermodynamic data from one of several sources.
The quality of thermodynamic data also determines the reliability of any speciation modeling. Filella and May (2003, 2005) collected and evaluated thermodynamic data for low molecular mass aqueous species of Sb(III) and Sb(V), resulting in a number of thermodynamically consistent equilibrium constants for some key equilibria. This evaluation identified some major deficiencies that limit the reliability of speciation modeling of antimony:
Some speciation models also include speciation forms which are not known to occur naturally (e.g. solid Sb(OH)3), or include a metastable solid speciation form such as Sb2O5(s). Taking the latter speciation phase into consideration, for instance, results in a misleading picture of the mobility of Sb(V) under strongly oxidizing conditions: this is one of the main elements that some authors have considered antimony to be highly mobile in the supergene environment.
A way to circumvent the limitations of speciation modeling could be direct (field) measurements. Methods exist that allow the determination of the Sb-fraction for both valence states. Unfortunately, obtaining information on the exact speciation phase is not always possible; for example, the complexing ability of the mobile-phase during a chromatographic run does alter the original species. Common sample treatment procedures (e.g. drying and grinding of soil) may also alter the speciation phases. In addition, current techniques are not sensitive enough to allow for possible antimony-organic complexes to be detected in natural environmental samples. Filella et al. (2009) also noted that most of the methods being developed for direct speciation measurement are never applied beyond the measurements that accompany the original publication.
Mobility and distribution of antimony in the environment
The mobility of antimony inthe evironment is limited by secondary mineral precipitation (Diemar et al., 2009; Filella and Williams, 2012; Roper et al., 2012), and partitioning to metal (hydr)oxides of Al, Fe, and Mn (Thanabalasingam and Pickering, 1990; Rakshit et al., 2011; Xu et al., 2011), clay minerals (Xi et al., 2010, 2011; Ilgen and Trainor, 2012), and humic acids (Pilarski et al., 1995; Tighe et al., 2005). These sorption and co-precipitation processes are the main pathways for Sb-removal from the water column to the sediment phase (Ashley et al., 2003; Beauchemin et al., 2012; Ritchie et al., 2013). The decrease in bioavailable antimony in water by oxic sediments is not a permanent decrease: the adsorption on the hydrous oxides is dependent on both pH and oxic condition (which may change). This is largely due to the redox cycling of iron (oxy) hydroxide precipitates with which antimony may be associated. In addition, antimony may become bioavailable to organisms inhabiting the sediment through ingestion of the sediment. In anoxic sediment layers, in combination with the presence of sulphide, antimony forms soluble or insoluble stibnite, SbS2 -and Sb2S3(s), respectively, depending on pH. This may result in a larger decrease in bioavailable antimony, when compared to the oxic part of the sediment. An example of natural attenuation of Sb is provided by Resongles et al. (2013). Reduction of Sb-levels in mine drainage waters occurred as a result of sorption processes to e.g. ochre precipitates and Fe/Mn oxides. The authors concluded that sorption of Sb(V) to pure Fe hydroxides appeared to be strong, but nevertheless less than that of Sb(III) to Fe and Mn (hydr)oxide surfaces.
Aqueous concentrations of trivalent antimony are typically limited by the Sb2O3 phases sénarmontite (to 1.2 µM) and valentinite (to 10 µM) (Zotov et al., 2003). Roméite (Ca2Sb2O7) is the typical limiting phase for the pentavalent phase (Roper et al., 2012), with a reported aqueous concentration of 0.33 ± 0.1 µM Sb(V) for roméite (Diemar et al., 2009).
Due to these solubility constraints, antimony is also considered relativelyimmobile in soil environments (Filella et al., 2002a; Knechtenhofer et al., 2003; Diemar et al., 2009; Reimann et al., 2010). Filella et al. (2006) stated that a very low percentage of antimony in soil is extracted by using mild extractants. When sequential extraction procedures are applied, some antimony is present in the ‘iron oxide fraction’ but most is present in the so-called residual fraction. Largely on the basis of such results, it has been established, and is generally accepted, that antimony is essentially immobile in soils. The speciation of Sb in real environmental soil samples can be defined by X-ray absorption spectroscopy (XAS); it should be noted, however that this method is only applicable when Sb-levels are sufficiently high, (i.e., soils affected by mining/tailings). The form in which antimony is present in such soils might be very different to that in non-polluted ones. Nevertheless, studies with soil samples from an area that was affected by an antimony smelter (Takaoka et al., 2005) an antimony mine pit (Mitsonubu et al. 2006), or soil samples from shooting ranges (Scheinost et al., 2006) found that antimony was present exclusively as Sb(V) and that the solid Fe3 +-hydroxide was the likely host phase (Filella et al., 2009). Uncertainty remains as to why Sb(III)-containing solid phases are not observed in soils with corroding Sb-containing bullets investigated up to date (Scheinost et al., 2006; Ackermann et al., 2009), while they are commonly observed as a result of stibnite oxidation, e.g. sénarmontite and valentinite (Roper et al., 2012), and during Sb(0) oxidation in laboratory studies (Linarez Pérez et al., 2010). With regard to Sb in the terrestrial compartment, Ilgen et al. (2014) concluded that the absence of trivalent Sb in soils was indicative of rapid Sb(III) oxidation to Sb(V) under surface soil conditions.
pH also determines the sorption of antimony to soil particles. The largest effect of pH on sorption seems to be around pH 3 - 4, with decreasing sorption at higher pH values. The effect of pH as such is probably less important when compared to the effect of the hydrous oxides. The effect of pH on antimony mobility seems to be via the influence of hydrous oxides on the valence of antimony and the solubility of the antimony compound, and also via the increasing negative charge of the soil at increasing pH (and hence, weaker sorption of the negatively charged Sb(OH)6-).
Conclusions:
Additional information
Information on Registered Substances comes from registration dossiers which have been assigned a registration number. The assignment of a registration number does however not guarantee that the information in the dossier is correct or that the dossier is compliant with Regulation (EC) No 1907/2006 (the REACH Regulation). This information has not been reviewed or verified by the Agency or any other authority. The content is subject to change without prior notice.
Reproduction or further distribution of this information may be subject to copyright protection. Use of the information without obtaining the permission from the owner(s) of the respective information might violate the rights of the owner.
