Registration Dossier
Registration Dossier
Data platform availability banner - registered substances factsheets
Please be aware that this old REACH registration data factsheet is no longer maintained; it remains frozen as of 19th May 2023.
The new ECHA CHEM database has been released by ECHA, and it now contains all REACH registration data. There are more details on the transition of ECHA's published data to ECHA CHEM here.
Diss Factsheets
Use of this information is subject to copyright laws and may require the permission of the owner of the information, as described in the ECHA Legal Notice.
EC number: 215-150-4 | CAS number: 1306-38-3
- Life Cycle description
- Uses advised against
- Endpoint summary
- Appearance / physical state / colour
- Melting point / freezing point
- Boiling point
- Density
- Particle size distribution (Granulometry)
- Vapour pressure
- Partition coefficient
- Water solubility
- Solubility in organic solvents / fat solubility
- Surface tension
- Flash point
- Auto flammability
- Flammability
- Explosiveness
- Oxidising properties
- Oxidation reduction potential
- Stability in organic solvents and identity of relevant degradation products
- Storage stability and reactivity towards container material
- Stability: thermal, sunlight, metals
- pH
- Dissociation constant
- Viscosity
- Additional physico-chemical information
- Additional physico-chemical properties of nanomaterials
- Nanomaterial agglomeration / aggregation
- Nanomaterial crystalline phase
- Nanomaterial crystallite and grain size
- Nanomaterial aspect ratio / shape
- Nanomaterial specific surface area
- Nanomaterial Zeta potential
- Nanomaterial surface chemistry
- Nanomaterial dustiness
- Nanomaterial porosity
- Nanomaterial pour density
- Nanomaterial photocatalytic activity
- Nanomaterial radical formation potential
- Nanomaterial catalytic activity
- Endpoint summary
- Stability
- Biodegradation
- Bioaccumulation
- Transport and distribution
- Environmental data
- Additional information on environmental fate and behaviour
- Ecotoxicological Summary
- Aquatic toxicity
- Endpoint summary
- Short-term toxicity to fish
- Long-term toxicity to fish
- Short-term toxicity to aquatic invertebrates
- Long-term toxicity to aquatic invertebrates
- Toxicity to aquatic algae and cyanobacteria
- Toxicity to aquatic plants other than algae
- Toxicity to microorganisms
- Endocrine disrupter testing in aquatic vertebrates – in vivo
- Toxicity to other aquatic organisms
- Sediment toxicity
- Terrestrial toxicity
- Biological effects monitoring
- Biotransformation and kinetics
- Additional ecotoxological information
- Toxicological Summary
- Toxicokinetics, metabolism and distribution
- Acute Toxicity
- Irritation / corrosion
- Sensitisation
- Repeated dose toxicity
- Genetic toxicity
- Carcinogenicity
- Toxicity to reproduction
- Specific investigations
- Exposure related observations in humans
- Toxic effects on livestock and pets
- Additional toxicological data
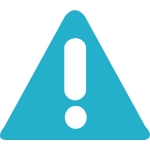
Endpoint summary
Administrative data
Link to relevant study record(s)
- Endpoint:
- basic toxicokinetics in vivo
- Type of information:
- experimental study
- Adequacy of study:
- supporting study
- Study period:
- Not applicable
- Reliability:
- 2 (reliable with restrictions)
- Rationale for reliability incl. deficiencies:
- other: :
- Remarks:
- though performed according to OECD guidelines, the study displayed some serious restrictions. First, there was no comparison with controls animals while background cerium levels in these animals were observed by other authors. Moreover, there were only 3 animals per groups and a high variability (as standard deviation (SD)) for almost all results. In addition, results were expressed as µg Ce/kg organ weights but without any precision on whether wet of dry tissue were concerned. Furthermore, organ weights were not measured in the study, data came from another study. There were imprecision regarding the total inhaled dose (i.e., not clear if data reported in the article related to single or repeated exposure), no clear data regarding detection limits from the apparatus used (ICP-MS and ICP-AES) in the study. The design of repeated exposures was not equivalent in term of exposure and seemed not well designed. At last, the interpretation of data could be confusing as the way results were expressed changed for each figure.
- Objective of study:
- distribution
- excretion
- other: accumulation
- Qualifier:
- according to guideline
- Guideline:
- other: OECD Guideline 412 (Repeated Dose Inhalation Toxicity: 28/14-Day)
- Deviations:
- yes
- Principles of method if other than guideline:
- - Nose-only inhalation of an aerosol of nanometric cerium dioxide (nano-CeO2) or micrometric CeO2 (bulk) in rats; sacrifice of animals within one hour after the last exposure and 48 or 72 h after the end of exposures
- Distribution in blood and tissue: inductively coupled plasma-mass/ -atomic emission spectrometry (ICP-MS/-AES)
- Modelisation of CeO2 deposition in rat lung: Multiple-Path Particle Dosimetry Model (MPPD) and calculations using MMAD, CMD, GSD, CeO2 mass concentration - GLP compliance:
- not specified
- Remarks:
- The GLP status was not specified in the article.
- Radiolabelling:
- no
- Species:
- rat
- Strain:
- Wistar
- Sex:
- male
- Details on test animals or test system and environmental conditions:
- TEST ANIMALS
- Source: Charles River (Germany)
- Age at study initiation: 6-week old
- Weight at study initiation: 212 ± 14 g
- Fasting period before study: None according to OECD dossier
- Housing: By group of 2 or 5 animals in standard laboratory cage / individually housed during exposure periods
- Individual metabolism cages: No data available
- Diet: Ad libitum, except during exposure
- Water: Ad libitum, except during exposure
- Acclimation period: At least 5 days
ENVIRONMENTAL CONDITIONS
- Temperature: 22 ± 2°C of air entering the exposure unit
- Humidity: 30-70% in the exposure unit
- Air changes: No data available
- Photoperiod: Mentioned as controlled but not documented
IN-LIFE DATES: No data available - Route of administration:
- inhalation: aerosol
- Vehicle:
- unchanged (no vehicle)
- Details on exposure:
- TYPE OF INHALATION EXPOSURE: nose only
GENERATION OF TEST ATMOSPHERE / CHAMPER DESCRIPTION
- Exposure apparatus: The apparatus was composed of nose-only exposure units consisting of a cylindrical column, surrounded by a transparent hood. The test atmosphere was introduced at the top of the central column and was exhausted at the bottom of ca. 50-L exposure unit. Each column included four rodent tube sections of 20 ports each for animal exposure.
- Method of holding animals in test chamber: Animals were secured in plastic holders (Battelle) and positioned radially through the outer cylinder around the central column introducing the test atmosphere.
- Source and rate of air: At least 1 L/min for each rat (monitored at least 3 times a day)
- Method of conditioning air: No data available
- System of generating particulates/aerosols: To generate the test atmosphere, CeO2 was aerosolised using a dust feeder, a Venturi and a jetmill. The generated test atmosphere was directed to the top inlet of the exposure unit and at the bottom of the chambers the test atmosphere was exhausted.
- Method of particle size determination: Particle size distributions (as well as particle number counts) were measured daily during the experimental period with a scanning mobility particle sizer (SMPS) and an aerodynamic particle sizer (APS).
- Treatment of exhaust air: No data available
TEST ATMOSPHERE
- Particle size distribution, MMAD (Mass median aerodynamic diameter) / GSD (Geometric st. dev.): See in Table 1 below, in "other information on materials and methods"
- Other: The mass concentration was determined at least 6 times daily during the experimental period using gravimetric analysis. - Duration and frequency of treatment / exposure:
- 6 hours per day for up to 28 days with a number of exposures (6h/expo.) ranging from 1 to 20
Sacrifice 1, 48 and 72 h after the end of the last administration (see in Table 2 below, in "other information on materials and methods") - Dose / conc.:
- 10.79 mg/m³ air (analytical)
- Remarks:
- for NM-211
basis: mean mass concentration (equivalent particle number concentration of 1.79 ± 0.42 x 10E6 particles/cm3 or total estimated inhaled dose of 0.83 mg) - Dose / conc.:
- 19.95 mg/m³ air (analytical)
- Remarks:
- for NM-212
basis: mean mass concentration (equivalent particle number concentration of 1.1 ± 0.70 x 10E6 particles/cm3 or total estimated inhaled dose of 1.54 mg) - Dose / conc.:
- 55 mg/m³ air (analytical)
- Remarks:
- for NM-213
basis: mean mass concentration (equivalent particle number concentration of 0.68 ± 0.46 x 10E6 particles/cm3 or total estimated inhaled dose of 4.24 mg) - No. of animals per sex per dose / concentration:
- 3 per group
- Control animals:
- not specified
- Details on study design:
- - Dose selection rationale: Geraets L et al. intentionally chose to aerosolise the particles from a powder to mimic realistic exposure scenarios, rather than trying to get an aerosol consisting of only single primary particles. The particle number concentrations (in the test atmosphere were targeted to be the same for each CeO2 type: 1.79 ± 0.42 10E6 particles/cm3 for NM-211, 1.1 ± 0.70 10E6 particles/cm3 for NM-212 and 0.68 ± 0.46 x 10E6 particles/cm3 for NM-213.
- Satellite group: For each CeO2, a recovery group was also included in the study (group IV) in order to study elimination of CeO2.
- Deposition Model calculation:
In order to estimate the dose deposited in the rat lung upon inhalation the multiple path particle dosimetry model (MPPD v 2.1.1) was used. The deposition fractions in the head, tracheobronchial and pulmonary region were calculated. Geraets L et al. thus used the default parameters of the model for rats, i.e., a forced respiratory capacity of 4 mL, head volume of 0.42 mL, nasal breathing, tidal volume of 2.1 mL and a breathing frequency of 102 per min. The inspiratory fraction was 0.5 and no pause was entered. Calculations were done using the measured MMAD, count median diameter (CMD), geometric SD, the mass concentration and a density of 7.65 g/cm3.
More detailed calculations were performed to estimate deposition in the olfactory epithelium because deposited particles may directly translocate to the brain, by passing the blood brain barrier, according to the authors. A detailed anatomically accurate computational fluid dynamics (CFD) model of the rat nasal passages was used for these calculations. The CFD model originally developed by Kimbel et al. (1997) and was subsequently smoothed using medical imaging software to provide a more realistic anatomic form according to Schroeter et al. (202). Particles sizes from 1 nm to 5 µm were simulated and nasal and olfactory deposition fractions were predicted for each particle size. - Details on dosing and sampling:
- At different time points during and after the study, tissue and blood samples were collected. For each CeO2, necropsy was performed within one hour after finishing the final exposure for all groups (I, II, III). Furthermore, blood and tissues from rats of the recovery group (IV) were sampled 48 or 72 h after the last exposure instead of within 1 h after exposure.
- BLOOD / ABSORPTION:
During the study, blood samples were taken at various time points after a single or repeated exposure. Blood sampling was performed in most cases well within the first hour after exposure, but also at post-exposure periods of up to 67 hours. Blood was sampled by orbital puncture under O2/CO2 anaesthesia in EDTA-containing tubes and frozen (below -18°C). Blood samples were digested with suprapure nitric acid. Total Ce content was then measured by inductively coupled plasma-mass spectrometry (ICP-MS).
- TISSUES / DISTRIBUTION:
For tissue sampling, rats were sacrificed while under ether anaesthesia, and tissues (lung, liver, spleen, kidney, testicles, epididymis and brain) were removed and frozen (below -18°C). Tissue samples were digested with a mixture of suprapure nitric, sulphuric and perchloric acids (2:1:1). After oxidation the mineral acids were evaporated and the residue was taken up with suprapure hydrochloric acid. Total Ce content in the lung tissue samples was measured by ICP-optical emission spectrometry (ICP-AES), whilst total Ce content in the other tissue samples was measured by ICP-MS.
- DATA EVALUATION:
The measured Ce tissue levels were converted into CeO2 tissue levels by multiplying the former with the ratio of the molecular masses of CeO2 and Ce (i.e., 1.23), thus assuming that the deposited and distributed CeO2 was still present as particles. This assumption was considered appropriate since results of solubility tests indicated that the solubility of NM-211 and NM-212 was extremely poor.
As the mass concentrations of the different nano-CeO2 were not similar because particle number concentrations were targeted to be the same, CeO2 tissue concentrations were normalised for the total inhaled dose in order to study particle-dependent differences in tissue concentrations. First, the absolute amount of CeO2 per tissue was calculated using organ weights.
As no information on the total organ weights of rats in the present study was available, data on organ from a different rat study were used (Lankveld et al., 2010). Rats used in the alternative study were similar to the animals used by Geraets L et al. with respect to strain, sex, age and bodyweight. Second, the total inhaled dose of CeO2 was calculated using the following formula: tidal volume x breathing frequency x exposure concentration x exposure duration (analogous to parameter settings of the MPPD model; the tidal volume was 2.10 mL and breathing frequency was 102 per min). Finally, the absolute amounts of CeO2 in tissue were normalized by expressing these amounts as percentage of the total inhaled dose CeO2 (i.e., 1.54, 0.83 and 4.24 mg CeO2 for NM-212, NM-211 and NM-213, respectively).
A tissue-specific detection limit was indicated to be < 5 µg/kg but there was no precision whether this data related to all tissue or to blood only and whether this value concerned ICP-MS or ICP-AES. - Statistics:
- Data on CeO2 contents in tissues were not normally distributed. The non-parametric Mann-Whitney U test was used to evaluate the effect of particle size on CeO2 tissue content, to study potential tissue-dependent differences in CeO2 levels and to assess the potential effect of number of exposures and recovery period on the CeO2 tissue levels. Two-tailed P values of 0.05 or less were considered statistically significant.
- Preliminary studies:
- No data available
- Details on absorption:
- Geraets L et al. stated that cerium (Ce) concentration in blood after single and repeated exposure to the tested nano-CeO2 (and micro-CeO2) was below the detection limit (< 5 µg/kg) for most samples (data not shown). According to Geraets L et al., this result indicated a fast distribution from blood to tissues. This result could also indicate a poor translocation of CeO2 to extrapulmonary tissues. Of note that there was no indication on the Ce concentration in blood of control rats.
- Details on distribution in tissues:
- After single and repeated exposure, Ce could be detected in all investigated organs, although substantial higher levels were measured in the lungs. The total amount of CeO2 measured in the tissues was far below the inhaled dose. According to Geraets L et al., Ce tissue levels in control rats were very low and very close to and partly below the tissue-specific detection limits (data not shown). However, the authors did not provide any numerical data on the tissue-specific detection limits; thus, Ce levels in control rats could not be extrapolated.
- SINGLE EXPOSURE (see in Table 3):
Though small differences in the pulmonary deposition efficiencies of CeO2 were observed, no obvious size-dependent differences in the pulmonary deposition of nano-CeO2 occurred. The percentage of inhaled CeO2 measured in lung tissue for NM-212 (5.6%) was significantly lower than the lung content for NM-211 (9.4%) and NM-213 (12.2%). According to the authors, these data indicated that NM-212 particles were less efficiently deposited in the lung as compared with NM-211 and NM-213 particles. However, the statistical analysis could be questionable as the comparison was only performed between NM-211 and NM-212 data, NM-213 and control data were not included. Moreover, when CeO2 content in lungs was recalculated as total amount accumulated, it was noted that lung CeO2 contents were similar between rats exposed to NM-211 and those treated with NM-212: 80 µg for NM-211 and 90 µg for NM-212.
CeO2 detected in the extrapulmonary tissues after a single exposure was less than 0.2% of the inhaled dose for all CeO2 particles, at least in the liver and even well below 0.05% in other organs. Unlike the deposition in the lung, the extrapulmonary CeO2 tissue levels for NM-212 were slightly (though not statistically significant) increased as compared to the extrapulmonary tissue levels of rats exposed to NM-211 and NM-213, indicating a moderately better distribution to extrapulmonary organs according to Geraets L et al. It has to be noted that high variability was measured among the 3 animals dosed with NM-212, thus preventing any firm conclusion on possible difference. Furthermore there was no data from controls reported in the article.
In order to study tissue-dependent differences in CeO2 concentrations, tissue CeO2 levels, measured by ICP-AES and -MS, were expressed as µg per kg tissue. After a single exposure, NM-211, NM-212, as well as NM-213 levels in lung tissue were significantly higher (at least 1000x) than in the other investigated tissues. NM-212 particles were distributed to the extrapulmonary tissues with an order of concentration of testicles = kidney < spleen = liver < brain < epididymis. Except for a slightly reduced distribution of NM-211 to testicles, epididymis, and brain, no clear differences in distribution pattern to the various extrapulmonary organs were observed after a single exposure to NM-211 particles. Of note that NM-213 particles were distributed to extrapulmonary tissues with an order of concentration of testicles < kidney < liver < spleen < brain < epididymis.
It should be noted that CeO2 concentrations were recalculated by the authors using tissue weights obtained on control animals from previous studies as these data were not generated by Geraets L et al. Furthermore, depending on how results were expressed (e.g., % of inhaled dose, total amount, tissue concentration per tissue weight), the interpretation could differ. Indeed, when CeO2 contents were recalculated as total accumulated amount, it was found that, except for liver, less than 1 µg of CeO2 was accumulated in each extrapulmonary organ collected, regardless of CeO2 type. As there was no data available on control rats, it was not clear whether such very low contents really reflected an accumulation of CeO2 following inhalation exposure or whether such levels of CeO2 corresponded to a background content which could be find in control rats. Indeed, Yokel R et al. (2012) measured background levels of CeO2 in urine (0.038 µg) and faeces (1.04 µg) in non-treated rats, probably due to food contamination. Moreover, expressed as total estimated content, CeO2 particles were distributed to tissues with a different order of concentration: spleen < epididymis < kidney < testicles < brain < liver < lung.
In addition, some of the data available (based on 3 animals only) displayed a very high variability between treated animals in terms of CeO2 accumulation, notably the data on NM-212. This could raise questions about the relevance of the number of animals included in each group for instance.
- REPEATED EXPOSURE (see in Table 4):
After multiple exposures, differences in NM-212 distribution to the various extrapulmonary organs were less than after a single exposure, though testicles levels remained the lowest. Lung CeO2 levels were the highest of all investigated tissues for both NM-212 and NM-211 (from 400 to 1250 mg Ce/kg tissue). Except for a slightly reduced distribution to testicles, epididymis, and brain, no clear differences in distribution pattern to the various extrapulmonary organs were observed after repeated exposures.
CeO2 tissue levels were normalized for the tissue levels after single exposure in order to evaluate potential accumulation after repeated exposure. Repeated exposure to all nano-CeO2 was found to result in significant increasing CeO2 concentrations in lungs and liver, indicating that accumulation occurred in these tissues. This was most evident and significant in lung tissue but less clear in the other sampled tissues. According to the authors, increasing the number of repeated exposures was found to result in higher fold of tissue accumulation for rats exposed to NM-212 (and NM-213), indicating that steady state was not yet reached after 11 exposures. Significant increases in CeO2 lung (NM-212 and NM-213) and liver (NM-212) levels could be observed. However, according to the corresponding figure, significant increases in CeO2 lung were seen for all CeO2 and a significant elevation of CeO2 content in liver was observed for NM-211 and NM-213 followed by a decrease in liver of rats from the recovery group treated with NM-212. Moreover, after single and repeated exposures, the accumulation pattern of NM-212 differed from the one of NM-211: based on the related figure, relevant CeO2 accumulation was only seen in lungs for NM-212, while the accumulation of NM-211 was evident in lung and all extrapulmonary tissues expect for epididymis.
Geraets L et al. noted that potential exposure via the oral route following inhalation exposure of particles might also contribute to extrapulmonary tissue deposition.
Moreover, it has to be noted that the authors pointed out a number of limitations in their study:
* the data were insufficient to predict the biodistribution in rodents and humans since the study included a limited set of time point to get rough perspective on the biodistribution of CeO2;
* very small amounts of cerium were detected in organs other than the lung; and thus it could not be ruled out that contamination occurred during autopsy of organs, for instance, due to the perfusion of the organs. - Details on excretion:
- The elimination half-life could not be reliably determined by the authors. Indeed, the elimination of NM-212 and NM-213 particles from the investigated tissues was slow, with, in almost all cases, insignificant amounts of CeO2 eliminated within 72 h (see in Table 4). Significant decrease in brain cerium levels was observed in NM-213 exposed rats. NM-212 exposed rats showed significant decreases in lung and liver Ce levels after recovery. A decreasing trend was observed in epididymis and spleen from rats exposed to NM-212 and NM-213. Elimination of NM-211 particles appeared to be even slower.
Of note that the recovery period seemed to be too short to allow assessing accurately the elimination of CeO2, thus potentially explaining that the authors could not determine the elimination half-life of CeO2. Moreover, CeO2 elimination was estimated based only on CeO2 contents in the investigated tissues; CeO2 quantification in faeces or urine was not included. In addition, it seemed difficult to draw any conclusion on CeO2 elimination since the related data displayed a high variability (i.e., high standard deviations (SD)). The low number of animals included in each group could explain these high SD. Further, there was no data on CeO2 contents in control rats; these data would have been interesting for comparison purpose. At last, it could not be ruled out that an elimination of inhaled CeO2 occurred via exhalation during, or shortly after the exposure period and/or that a rapid elimination of deposited CeO2 occurred via the mucociliary escalator of the bronchia and conducting airways. - Metabolites identified:
- not measured
- Bioaccessibility (or Bioavailability) testing results:
- No data available
- Conclusions:
- After both single and repeated exposures, the highest levels of CeO2 were found in lung tissues. No cerium was detected in the blood of either control or treated rats. Distribution of the inhaled CeO2 particles to various extrapulmonary tissues was also observed, but largely in a lower extent (µg/kg tissue) than in the pulmonary tissues (mg/kg tissue).Though small differences in pulmonary deposition efficiencies of CeO2 were observed, no consistent relationship between the primary particle size, MMAD or surface area and pulmonary deposition and extrapulmonary tissue distribution was noted. Either the aggregates were not broken up by physiological fluids and remained intact during distribution, or nanoparticles and microparticles distributed similarly. Furthermore, tissue clearance of the particles seemed to be slow, and insignificants amounts of CeO2 were eliminated within the 48-72 h following the end of exposure period. No differences among the tested CeO2 were seen in terms of pathology or other markers of toxicity.
=> Interpretation of results: low bioaccumulation potential, with no size effect of particles - Executive summary:
Geraets L et al. (2012) investigated absorption, tissue distribution, accumulation, and elimination of nanometric cerium dioxide (nano-CeO2) with various physico-chemical properties during and after a 28-day inhalation study in rats. This study was conducted within the context of the OECD Working Party on Manufactured Nanomaterials Sponsorship Program. Of note that the study was designed to compare nano-CeO2 to bulk cerium dioxide (micro-CeO2 NM-213).
The tested CeO2 were physico-chemically characterised:
Parameters
Results
Methods
Antaria - NM-211
Umicore - NM-212
Sigma-Aldrich - NM-213
Physical state
Solid
Solid
Solid
-
Molecular weight
172.11
172.11
172.11
No data
Primary particle size
5 to 10 nm
13.0 ± 3.2 nm
44.9 ± 14.6 nm
12 nm
40 nm
27.3 ± 13.6 nm
28.4 ± 10.4 nm
29 nm
< 5000 nm
Sample too large for TEM measurement (> 500 nm)
615.3 ± 430.5 nm
213 nm
Supplier’s data (nominal)
TEM (Feret diameter)
SEM (Feret diameter)
Diameter calculated from BET surface area (nm) [B]
Particle size distribution - MMAD
1.02 ± 0.04 µm
(1.82) [A]
1.17 ± 0.34 µm
(2.07) [A]
1.40 ± 0.11 µm
(1.64) [A]
APS
Count median diameter (CMD)
0.21 ± 0.03 µm
(1.76) [A]
0.25 ± 0.07 µm
(1.78) [A]
0.34 ± 0.06 µm
(1.52) [A]
SMPS
Stability
Aggregation
Aggregation
Aggregation
-
Specific surface area
63.95 ± 0.30 m²/g
27.15 ± 0.19 m²/g
3.73 ± 0.01 m²/g
BET
Analytical purity / impurities
99.5% purity
99.95% purity
99.5% purity
No data
Particle number concentration
1.79 ± 0.42 x 10E6 particles/cm3
1.1 ± 0.70 x 10E6 particles/cm3
0.68 ± 0.46 x 10E6 particles/cm3
SMPS
Solubility
Extremely poor in water at neutral pH
Extremely poor in water at neutral pH
Extremely poor in water at neutral pH
No data
Assumed density
* 7.65 g/cm3 according to the authors
* 7.13 g/mL at 25°C according to OECD dossier
* 7.65 g/cm3 according to the authors
* 7.13 g/mL at 25°C according to OECD dossier
* 7.65 g/cm3 according to the authors
* 7.13 g/mL at 25°C according to OECD dossier
-
Mean mass concentration
10.79 ± 0.82 mg/m3
19.95 ± 13.21 mg/m3
55.00 ± 6.20 mg/m3
TGA
Total estimated inhaled dose [C]
0.83 mg
1.54 mg
4.24 mg
Calculation for single exposure
Results are mean ± standard deviation (SD) of multiple measurements.
[A] geometric standard deviation
[B] calculated using the formula: d = 6 / (surface area x density)
[C] calculated using the formula: tidal volume x breathing frequency x exposure concentration x exposure duration (analogous to parameter settings of the MPPD model; the tidal volume was 2.10 mL and breathing frequency was 102 per min).
Powder aerosolization resulted in comparable mass median aerodynamic diameter (MMAD): 1.17 µm for NM-212, 1.02 µm for NM-211 and 1.40 µm for NM-213, meaning that particles aggregated in the test atmosphere.
Male Wistar rats (3/gp) were exposed to CeO2 via inhalation route (nose-only), 6 h/day for 1 to 28 days with a number of exposures ranging from 1 to 20. Moreover, recovery groups were included in this study which consisted in sacrifice of rats 48 or 72 h instead of within 1 h after the last exposure. Though mentioned in the publication, there was no clear description on control animals. Particle concentrations were targeted to be the same: 1.79 x 10E6 particles/cm3 for NM-211, 1.1 x 10E6 particles/cm3 for NM-212, and 0.68 x 10E6 particles/cm3 for NM-213. However, the determination of CeO2 mass concentrations by thermogravimetric analysis demonstrated that CeO2 concentrations were different: 10.79 mg/m3 for NM-211, 19.95 mg/m3 for NM-212, and 55.00 mg/m3 for NM-213.
Geraets L et al. observed that CeO2 concentration in blood after single and repeated exposure was below the detection limit (i.e., < 5 µg/kg) for most samples. The authors suggested that this was due to a fast distribution of CeO2 from blood to tissues.
After single exposure, approximately 5 to 10% of the inhaled dose was measured in lung tissue, as was also estimated by a multiple path particle dosimetry model (MPPD). Though small differences in pulmonary deposition efficiencies of CeO2 were observed, no consistent differences in pulmonary deposition between NM-211, NM-212, and NM-213 were observed. After a single 6-h exposure, each CeO2 sample was also distributed to extrapulmonary tissues with an order of concentration of spleen (< 0.006%) ≤ epididymis (< 0.015%) ≤ kidney (< 0.015%) ≤ testicles (< 0.02%) ≤ brain (< 0.04%) < liver (< 0.15%; as maximal values as percent of inhaled dose). No clear particle size- or surface area-dependent effect on pulmonary deposition and extrapulmonary tissue distribution was observed.
According to the authors, repeated exposure to CeO2 particles resulted in significant accumulation of the particles in lungs and extrapulmonary tissues.
In addition, tissue clearance was shown to be slow, and, overall, insignificant amounts of CeO2 were eliminated from the body within the 48- to 72-h post-exposure period. However, CeO2 elimination was estimated based only on CeO2 contents in the investigated tissues; CeO2 quantification in faeces or urine was not included. Moreover, it seemed difficult to draw any conclusion on CeO2 elimination given the very low but highly variable levels of CeO2 found in extrapulmonary organs and given the lack of data on control animals.
The authors suggested that the lack of correlation between primary particle size and pulmonary deposition or extrapulmonary distribution was likely due to the presence of aggregates rather than single nano-sized particles in the test atmosphere resulting in similar aerodynamic diameter of micro- and nano-CeO2 but with different surface characteristics. The authors mentioned that these findings did not support the hypothesis that specific surface area is a good predictor of either the pulmonary or extrapulmonary dose for adverse effects.
Modelisation of lung deposition using MPPD displayed results in agreement with the data obtained from the inhalation study: 7, 8, and 9% of inhaled NM-213, -212, -211, respectively, were expected to be deposited in the lungs. Based on deposition modelisation, the olfactory deposition of the tested CeO2 was expected to be minimal, even though the deposition in the head region was relatively high. Thus, the olfactory route did not seem to have contributed to the contents of CeO2 observed in the brain. Contrarily, this content could have been resulted from contamination during autopsy.
In addition, according to Geraets L et al., no differences among both nano-CeO2 and micro-CeO2 were seen in term of pathology or other markers of toxicity.
In conclusion, following single and repeated inhalation, the tested nano-CeO2 and micro-CeO2 were deposited and mainly accumulated in the lungs, irrespective of the primary particle size of each CeO2 tested. No clear effect of the primary particle size or surface area on pulmonary deposition and extrapulmonary tissue distribution was observed, probably due to presence of aggregates. The data available suggested a possible accumulation in extrapulmonary tissues but CeO2 contents in the investigated tissues were extremely low. Geraets L et al. appropriately mentioned that they could not rule out that a contamination of extrapulmonary tissues had occurred during autopsy, thus explaining the low but detectable amounts of CeO2 found in these organs. Furthermore, insignificant amounts of CeO2 were eliminated in 48-72h.
- Endpoint:
- basic toxicokinetics in vivo
- Type of information:
- experimental study
- Adequacy of study:
- supporting study
- Study period:
- Not applicable
- Reliability:
- 3 (not reliable)
- Rationale for reliability incl. deficiencies:
- other: :
- Remarks:
- the study was considered to meet generally accepted scientific principles. However, there were some restrictions regarding the level of details available on test animals (e.g., source, age, weight) and the physico-chemical characteristics of nano-CeO2; but, at the publication date, the scientific community required less physico-chemical endpoints in such articles. Nevertheless, although described in "Materials and Methods" section, toxicokinetic data on control animals were not provided in the publication. Thus, it was difficult to assess the biological relevance of CeO2 distribution in investigated tissues. Moreover, there was no data available regarding the experimental validation of the detection method, ICP-MS (e.g., limit of detection). In addition, results were sometimes confusingly reported regarding the route of exposure and only 3 animals per group were used.
- Reason / purpose for cross-reference:
- reference to same study
- Reason / purpose for cross-reference:
- reference to same study
- Objective of study:
- distribution
- excretion
- other: immune response, histopathology
- Qualifier:
- no guideline followed
- Principles of method if other than guideline:
- - Repeated oral administration (gavage) of a suspension of nanometric cerium dioxide (nano-CeO2) to mice; sacrifice of animals 1 week after the last administration
- Tissue distribution: Light (LM) and electron microscopy (EM), immuno-histochemistry (IHC) and inductively coupled plasma mass-spectrometry (ICP-MS)
- Immune responses: Counting of white blood cells using an automate blood counter
- Histopathological analysis: EM - GLP compliance:
- not specified
- Remarks:
- The GLP status was not specified in the article.
- Radiolabelling:
- no
- Species:
- mouse
- Strain:
- CD-1
- Sex:
- female
- Details on test animals or test system and environmental conditions:
- TEST ANIMALS
- Source: No data available
- Age at study initiation: 8-week-old
- Weight at study initiation, fasting period before study, housing, individual metabolism cages, diet, water, acclimation period: No data available
ENVIRONMENTAL CONDITIONS
No data available
IN-LIFE DATES: No data available - Route of administration:
- oral: gavage
- Vehicle:
- other: sterile phosphate-buffered saline (PBS) solution
- Details on exposure:
- PREPARATION OF DOSING SOLUTIONS: No data available
VEHICLE
- Justification for use and choice of vehicle: No data available
- Concentration in vehicle: 0.5 mg/kg in 100 µL of PBS
- Amount of vehicle: 100 µL of PBS
- Lot/batch no., purity: No data available
HOMOGENEITY AND STABILITY OF TEST MATERIAL: No data available - Duration and frequency of treatment / exposure:
- Once a week for 2 or 5 weeks (sacrifice 1 week after the last administration, and thus on weeks 3 and 6 of the study)
- Dose / conc.:
- 0 mg/kg bw (total dose)
- Remarks:
- (control)
- Dose / conc.:
- 0.5 mg/kg bw (total dose)
- Remarks:
- basis: nominal nano-CeO2 conc.
- No. of animals per sex per dose / concentration:
- 2-week exposure => n = 3 per group
5-week exposure => n = 3 per group
The study was repeated giving a total of six mice in each experimental and control group. - Control animals:
- yes, concurrent vehicle
- Details on dosing and sampling:
- PHARMACOKINETIC STUDY (Absorption, distribution, excretion)
Major organs (brain, heart, lung, liver, pancreas, spleen, stomach, small intestine, and large intestine) were collected 1 week after the last administration, harvested and placed in 10% buffered formaldehyde until ready to be examined. Tissues were later patted dry, weighed and placed in 70% nitric acid overnight to start the digestion process. Samples were then microwave digested in an Xpress Microwave Digester. The temperature was ramped to 200°C over 20 min and held there for another 20 min. Once the digestion was over, Ce levels were assessed using inductively coupled plasma-mass spectrometry (ICP-MS). Some tissue samples were then viewed for nano-CeO2 deposition using HRTEM at 300 keV.
OTHER
- HISTOPATHOLOGY and IMMUNE RESPONSE:
Sections of brain, heart, lung, liver, pancreas, spleen, stomach, small intestine, and large intestine were fixed in 10% buffered formaldehyde for 24 h. Tissues were then trimmed, processed, sectioned (5 µm), and stained with hematoxylin and eosin (H&E) for histopathology. The samples were then viewed for nano-CeO2 deposition using high-resolution transmission electron microscopy (HRTEM) at 60 or 300 keV.
Immediately before euthanasia of mice by cervical dislocation under isofluorane anaesthesia, blood was collected by retro-orbital bleed. Complete blood counts were performed for each mouse using an automate blood counter: haemoglobin (Hb) level, erythrocyte (RBC) count, total leukocyte (WBC) count, and differential leukocytes (lymphocytes, neutrophils, monocytes). Additionally, smears of cells were drawn on clean sides, fixed with methanol for 10 min, and then stained with May-Granwald-Giemsa (Sigma-Aldrich). At least 1000 cells were scored from each animal to determine the differential elements (lymphoid and erythroid cells). All counts were performed in quadruplicate and averaged for a final count. - Statistics:
- Data were analysed using one-way ANOVA with Tukey’s post analysis or Student’s t test. A P value ≤ 0.05 was considered significant.
- Preliminary studies:
- No data available
- Details on absorption:
- No data available
- Details on distribution in tissues:
- CD-1 mice orally exposed to nano-CeO2 (0.5 mg/kg) had negligible nano-CeO2 deposition in any organ with the exception of the lungs displaying detectable CeO2 levels (ca. 1 ± 1 µg Ce/g at the end of 5-week exposure). However, the CeO2 levels found in the lungs were most likely due to gavage procedure or slight aspiration, according to Hirst SM et al.
- Details on excretion:
- Nano-CeO2 was found mainly cleared through faeces, with an excretion rate of ca. 98%. There was no detectable nano-CeO2 in urine of any group (data not shown). Similar results were found in both 2- and 5-week studies.
- Metabolites identified:
- not measured
- Bioaccessibility (or Bioavailability) testing results:
- No data available
- Conclusions:
- Nano-CeO2 administered once a week for 2 or 5 weeks via oral route was mainly excreted through faeces and lacked evidence of tissue distribution, general toxicity and overt pathogenic side effects.
=> Interpretation of results: low bioaccumulation potential based on study results - Executive summary:
Hirst SM et al. (2011) investigated the distribution of nanometric cerium dioxide (nano-CeO2) in mice exposed orally.
The in-house synthesised nano-CeO2 had a primary particle size of 3-5 nm, a crystalline structure and a Ce(III) surface enrichment. Moreover, the suspension had a pH < 3.5 and agglomerates of 10-50 nm were observed in the nano-CeO2 suspension.
Female CD-1 mice (≥ 3/gp) were orally dosed once a week for 2 or 5 weeks with 0 (control) or 0.5 mg/kg nano-CeO2as a suspension. Control mice were treated with sterile PBS (vehicle). Animals were sacrificed one week after the last administration. A histological examination of major organs (brain, lungs, liver, spleen, kidneys and pancreas) was performed using light (LM) and electron microscopy (EM). Nano-CeO2 distribution was evaluated in organs using microscopy (LM, EM), immuno-histochemistry (IHC) and inductively coupled plasma-mass spectrometry (ICP-MS). Moreover, immune responses were investigated by counting white blood cells.
The authors demonstrated that mice excreted through faeces around 98% of the administered nano-CeO2 within 24 h. This was probably due to a lack of uptake by the gastro-intestinal system. The remaining nano-CeO2 was mainly found in the lungs of orally exposed mice (ca. 1 ± 1 µg Ce/g at the end of 5-week exposure) and this was most likely due to slight aspiration according to the authors. There was no distribution of nano-CeO2 in the heart and brain of any mice. Moreover, nano-CeO2 did not cross the brain blood barrier. In addition, nano-CeO2 showed no overt toxicity and no induction of immune responses.
In conclusion, the tested nano-CeO2 displayed the classical behaviour of poorly soluble particles when given by oral route: low bioaccumulation and high faecal excretion.
- Endpoint:
- basic toxicokinetics in vivo
- Type of information:
- experimental study
- Adequacy of study:
- supporting study
- Study period:
- Not applicable
- Reliability:
- 3 (not reliable)
- Rationale for reliability incl. deficiencies:
- other: :
- Remarks:
- the statistical analyses were insufficiently documented and some methods and results were not clearly described, which made it difficult to understand (e.g., no controls animals). Moreover, the physico-chemical characterisation was performed on non-radioactive nano-CeO2 instead of the radioactive nanoparticles which were the material administered to animals.
- Reason / purpose for cross-reference:
- reference to same study
- Objective of study:
- distribution
- excretion
- Qualifier:
- no guideline followed
- Principles of method if other than guideline:
- - Single oral administration of a suspension of nanometric cerium dioxide (nano-CeO2) in rats; sacrifice of animals 1, 3 and 7 d after administration
- Pulmonary retention, elimination half-life, extrapulmonary translocation and excretion: Radiotracer technique (i.e., radioactive nano-CeO2) - GLP compliance:
- not specified
- Remarks:
- The GLP status was not specified in this article.
- Radiolabelling:
- yes
- Species:
- rat
- Strain:
- Wistar
- Sex:
- male
- Details on test animals or test system and environmental conditions:
- TEST ANIMALS
- Source: Experimental Animal Center, Peking University (China)
- Age at study initiation: No data available
- Weight at study initiation: 190-210 g
- Fasting period: Deprivation before oral exposure and until 6 h after administration
- Housing: Individually in stainless steel cages
- Individual metabolism cages: No data available
- Diet: Ad libitum (commercial pellet diet)
- Water: Ad libitum (deionized water)
- Acclimation period: One week
ENVIRONMENTAL CONDITIONS
- Temperature: 20 ± 2°C
- Humidity: 50-70% relative humidity
- Air changes: No data available
- Photoperiod: 12 hrs dark / 12 hrs light
IN-LIFE DATES: No data available - Route of administration:
- oral: gavage
- Vehicle:
- other: Milli-Q ultrapure water
- Details on exposure:
- Three groups of rats were exposed to nano-CeO2 via oral gavage after 12 h fasting. Nano-CeO2 suspension (1 mg/mL in 1 mL) was introduced into the GI tract.
- Duration and frequency of treatment / exposure:
- A single exposure (sacrifice 1, 3 and 7 d after oral administration)
- Dose / conc.:
- 1 other: mg/mL
- Remarks:
- 0.1 mL of a suspension of 1 mg/mL
basis: nominal nano-CeO2 conc. - No. of animals per sex per dose / concentration:
- 6
- Control animals:
- no
- Details on dosing and sampling:
- PHARMACOKINETIC STUDY (Absorption, distribution, excretion)
The fasted state of exposed animals was continued until 6 h after administration. At 1, 3, and 7 d post-exposure, anaesthetized rats were sacrificed after 12 h fasting and the sample collection conducted was similar to the intratracheal instillation study.
RADIOACTIVITY DETERMINATION:
Nano-CeO2 biodistribution was determined by measuring the activities of 141Ce in samples using a photon detector with a relative detection efficiency of 30%. The content of nano-CeO2 was calculated by comparing the radioactivity in the sample to the reference standard. The data were expressed as the content in tissues or the percentage of the given dose. The pharmacokinetics of intratracheally instilled nano-CeO2 in the lung was evaluated using a first-order kinetics model. - Statistics:
- No data available
- Preliminary studies:
- No data available
- Details on absorption:
- The results of the gavage study suggested that nano-CeO2 could hardly be absorbed in the GI tract. The absorption of nano-CeO2 in the GI tract was barely discernible.
- Details on distribution in tissues:
- Though a 5-fold dose was introduced via gavage, the contents of nano-CeO2 in liver, spleen, blood and bone at day 1 post-exposure were about one order of magnitude less, comparing to the corresponding results at day 1 after intratracheal instillation (see data in ESR entitled "nano-CeO2 - biodistribution in rats - i.t. - V3 2010HeXi"). According to the figures available in the article, the highest contents of nano-CeO2 were found in stomach and intestine, the lowest in testicle and blood: blood < testicle < brain < lung < heart < spleen < kidney
- Details on excretion:
- Over 90% of the administered particles were excreted through faeces during the first day post-exposure and nearly 100% were excreted by day 3 post-exposure.
- Metabolites identified:
- not measured
- Bioaccessibility (or Bioavailability) testing results:
- No data available
- Conclusions:
- Orally administered, nano-CeO2 was barely absorbed in the GI tract; nearly all particles were excreted by day 3 post-exposure.
=> Interpretation of results: low bioaccumulation potential based on study results- Executive summary:
He X et al. (2010) employed the radiotracer technique to study the impact of nano-CeO2 uptake via gastrointestinal (GI) tract into rats exposed via oral route.
Radiolabelled nano-CeO2 was used in this study. The nanoparticles were synthesised from radioactive Ce(NO3)3 using a precipitation method. However, as the authors assumed that the radiolabelled and non-labelled nanoparticles had the same physico-chemical properties, only the non-radiolabelled nano-CeO2 were characterised. The insoluble and crystalline nano-CeO2 had a primary size of 6.6 nm, a hydrodynamic size of 12.8 nm, a specific surface area of 86.85 mg²/g and a zeta potential of +30.1 mV. He X et al. also tested the stability of non-radioactive nano-CeO2 suspended in various media. The results showed that nano-CeO2 agglomerated in all media (e.g., ultrapure water, or physiological saline solution), but adding serum or albumin reduced, or even prevented, the nano-CeO2 agglomeration.
Male Wistar rats (6/group) were exposed once to radiolabelled nano-CeO2 (1 mg/mL or 4.7 - 5.3 mg/kg bw) via oral gavage and sacrificed at 1, 3, and 7 d after exposure. Cerium content, based on radioactivity measurements, was quantified in blood, lungs, heart, liver, kidney, spleen, stomach, intestine, testicle, brain, left femur, skeletal muscle, faeces and urine.
In this gavage study, it was found that over 90% of the gavage dose was excreted during the first day and the administered dose was almost excreted completely (over 99%) within faeces during the first 3 days after administration. According to the figures available in the article, the highest contents of nano-CeO2 were found in stomach and intestine, the lowest in testicle and blood: blood < testicle < brain < lung < heart < spleen < kidney <muscle < bone < liver < intestine < stomach at days 1, 3, and 7. Nano-CeO2 was thus barely absorbed in the GI tract, though a large amount of nano-CeO2 was orally introduced. Moreover, the transit of particles through the GI tract did not seem to contribute to the increases of nano-CeO2 in the extrapulmonary organs.
In conclusion, the tested nano-CeO2 displayed the classical behaviour of poorly soluble particles: low bioaccumulation and high faecal excretion.
- Endpoint:
- basic toxicokinetics in vivo
- Type of information:
- experimental study
- Adequacy of study:
- supporting study
- Study period:
- Not applicable
- Reliability:
- 3 (not reliable)
- Rationale for reliability incl. deficiencies:
- other: :
- Remarks:
- there were some restrictions regarding the level of details available on test animals (e.g., age, weight, diet) and the physico-chemical characteristics of nano-CeO2. However, at the publication date, the scientific community required less physico-chemical endpoints in such articles. Moreover, this study did not correspond to a true toxicokinetics/biodistribution study but was rather an acute oral study in which levels of nano-CeO2 were measured in some organs. Furthermore, the levels of CeO2 reported into the lungs were not discussed regarding a possible reflux into the respiratory tract during gavage. At last, there was no data available regarding the experimental validation of the detection method, ICP-MS (e.g., limit of detection).
- Reason / purpose for cross-reference:
- reference to same study
- Objective of study:
- distribution
- Qualifier:
- according to guideline
- Guideline:
- other: as part of an acute oral toxicity study performed according to guidelines from Korea Food and Drug administration
- Deviations:
- not specified
- Remarks:
- (not possible to assess)
- Principles of method if other than guideline:
- - Single oral administration of a nanometric cerium dioxide (nano-CeO2) suspension in rats; sacrifice of animals on day 1, 7, and 14 after treatment
- Distribution: Inductively coupled plasma-mass spectrometry (ICP-MS) - GLP compliance:
- not specified
- Remarks:
- The GLP status was not specified in the article.
- Radiolabelling:
- no
- Species:
- rat
- Strain:
- Sprague-Dawley
- Sex:
- male
- Details on test animals or test system and environmental conditions:
- TEST ANIMALS
- Source: Orient-Bio Animal Company (Korea)
- Age at study initiation, weight at study initiation, fasting period, housing, individual metabolism cages, diet, water: No data available
- Acclimation period: Included but duration not stated
ENVIRONMENTAL CONDITIONS
- Temperature: 23 ± 1°C
- Humidity: 55 ± 5%
- Air changes: No data available
- Photoperiod: 12 hrs dark / 12 hrs light
IN-LIFE DATES: No data available - Route of administration:
- oral: gavage
- Vehicle:
- other: distilled water
- Details on exposure:
- PREPARATION OF DOSING SOLUTIONS:
Nano-CeO2 was suspended in distilled water and sonicated for 30 min before treatment.
VEHICLE
No data available
HOMOGENEITY AND STABILITY OF TEST MATERIAL:
No data available - Duration and frequency of treatment / exposure:
- A single exposure (sacrifice on day 1, 7, and 14 after treatment)
- Dose / conc.:
- 0 mg/kg bw (total dose)
- Remarks:
- (control)
- Dose / conc.:
- 100 mg/kg bw (total dose)
- Remarks:
- basis: nominal nano-CeO2 conc.
- Dose / conc.:
- 5 000 mg/kg bw (total dose)
- Remarks:
- basis: nominal nano-CeO2 conc.
- No. of animals per sex per dose / concentration:
- - 4 in control group
- 6 in treated group - Control animals:
- yes
- Details on dosing and sampling:
- PHARMACOKINETIC STUDY (Distribution)
- Tissues and body fluids sampled: Liver, kidney, spleen, lung, testicles and brain
- Time and frequency of sampling: Tissues were collected from rats of control group and high-dose treated group on day 1, 7 and 14 after treatment.- Other: The wet tissues were dehydrated using freeze-dryer and the freeze-dried tissues were digested in the solution of HNO3 and H2O2 using microwave digestion system. Tissue concentration of cerium (Ce) was analysed using inductively coupled plasma-mass spectrometry (ICP-MS). - Statistics:
- Results of tissue distribution analysis were presented as the mean ± standard deviation (SD). The results of the chemically-treated groups were compared to those of the control group. The values were compared using the Student’s t-test, and levels of significance were represented for each result (p < 0.01).
- Preliminary studies:
- No data available
- Details on absorption:
- According to the authors, most of the administered nano-CeO2 was not absorbed into blood stream.
- Details on distribution in tissues:
- When rats were treated with both doses of nano-CeO2 and were sacrificed on day 1, nano-CeO2 accumulation in rat tissues seemed to occur mainly in lungs. The increase was statistically significant only for lung tissue and for both treatment groups (ca. 35.0 µg/g tissue at 5000 mg/kg and ca. 1.5 µg/g at 100 mg/kg). Thus, according to the authors, the lungs seemed to be the main target organ of nano-CeO2 by oral administration. Though significant, the elevated levels observed in lungs from animals treated with 5000 mg/kg nano-CeO2 were decreased on days 7 and 14 (ca. 15 and ca. 4.5 µg/g, respectively) in a time-dependent manner. In the low-dose treated group, the CeO2 levels in lungs decreased on day 7 (ca. 0.6 µg/g) and returned to control level by day 14 post-exposure (ca. 0.4 µg/g). No significant increase was observed in any of the other organs collected. According to the authors, the decrease in lung CeO2 level observed suggested that a lung clearance of nano-CeO2 occurred over time. However, such observations in lungs were not described in the other studies available on nano-CeO2 toxicokinetic behaviour following oral administration (He X et al., 2010; Kumari M et al., 2014; Molina RM et al., 2014); except for the study of Hirst SM et al. (2011) in which the authors detected Ce in lungs but claimed that lung CeO2 levels were most likely due to gavage procedure or slight aspiration. Thus, the fast removal of CeO2 from lungs described by Park EJ et al. could also suggest that some test item could have passed into the respiratory tract during the gavage could occurred. This could explain the pulmonary accumulation of nano-CeO2, although no or few administered nano-CeO2 was absorbed into blood stream according to the authors.
- Details on excretion:
- According to the authors, most of the administered nano-CeO2 was excreted through faeces. After oral administration, the faeces of the treated rats turned to be white which was the colour of CeO2 pigment.
- Metabolites identified:
- not measured
- Bioaccessibility (or Bioavailability) testing results:
- No data available
- Conclusions:
- Orally administered nano-CeO2 was barely absorbed in the gastro-intestinal tract but mainly excreted through faeces.
=> Interpretation of results: low bioaccumulation potential based on study results - Executive summary:
Park EJ et al. (2009) evaluated the tissue distribution of nanometric cerium dioxide (nano-CeO2) in rats exposed via oral route during an acute oral toxicity study.
In-house synthesised nano-CeO2 was used in this study. The crystalline nanoparticles displayed a cubic-like shape and a crystallite size of 30 nm. These nanoparticles were suspended in distilled water and sonicated 30 min prior to administration.
Male Sprague-Dawley rats (4 - 6/group) were administered a single nano-CeO2 dose of 0 (control), 100 or 5000 mg/kg by gavage and sacrificed on days 1, 7 and 14 after exposure. Tissue distribution analysis in liver, kidney, spleen, lung, testicles and brain was performed using inductively coupled plasma-mass spectrometry (ICP-MS).
When rats were treated with both doses of nano-CeO2 and were sacrificed on day 1, nano-CeO2 accumulation in rat tissues seemed to occur mainly in lungs. The increase was statistically significant only for lung tissue and for both treatment groups (ca. 35.0 μg/g tissue at 5000 mg/kg and ca. 1.5 μg/g at 100 mg/kg). Thus, according to the authors, the lungs seemed to be the main target organ of nano-CeO2 by oral administration. Though significant, the elevated levels observed in lungs from animals treated with 5000 mg/kg nano-CeO2 were decreased on days 7 and 14 (ca. 15 and ca. 4.5 μg/g, respectively) in a time-dependent manner. In the low-dose treated group, the CeO2 levels in lungs decreased on day 7 (ca. 0.6 μg/g) and returned to control level by day 14 post-exposure (ca. 0.4 μg/g). No significant increase was observed in any of the other organs collected. According to the authors, the decrease in lung CeO2 level observed suggested that a lung clearance of nano-CeO2 occurred over time. However, such observations in lungs were not described in the other studies available on nano-CeO2 toxicokinetic behaviour following oral administration (He X et al., 2010; Kumari M et al., 2014; Molina RM et al., 2014); except for the study of Hirst SM et al. (2011) in which the authors detected Ce in lungs but claimed that lung CeO2 levels were most likely due to gavage procedure or slight aspiration. Thus, the fast removal of CeO2 from lungs described by Park EJ et al. could also suggest that some test item could have passed into the respiratory tract during the gavage could occurred. This could explain the pulmonary accumulation of nano-CeO2, although most of the administered nano-CeO2 was not absorbed into blood stream but rather excreted through faeces according to the authors.
In addition, the authors described no systemic toxicity in rats orally exposed to 100 and 5000 mg nano-CeO2/kg: no mortality, no change in serum biochemistry and hematology, no histopathological lesion (see in "Acute Toxicity" section).
In conclusion, the tested nano-CeO2 displayed the classical behaviour of poorly soluble particles: low bioaccumulation and high faecal excretion.
- Endpoint:
- basic toxicokinetics in vivo
- Type of information:
- experimental study
- Adequacy of study:
- weight of evidence
- Study period:
- Not applicable
- Reliability:
- 2 (reliable with restrictions)
- Rationale for reliability incl. deficiencies:
- study well documented, meets generally accepted scientific principles, acceptable for assessment
- Remarks:
- ; but test not conducted under GLP conditions.
- Reason / purpose for cross-reference:
- reference to other study
- Reason / purpose for cross-reference:
- reference to other study
- Objective of study:
- distribution
- excretion
- other: biopersistence
- Qualifier:
- no guideline followed
- Principles of method if other than guideline:
- - Unique intravenous (i.v.) injection of a suspension of nanometric cerium dioxide (nano-CeO2) in rats; sacrifice of animals 1 and 20 hours and 1, 7, 30, or 90 days after injection
- Tissue distribution: Inductively coupled plasma mass spectrometry (ICP-MS) and electron microscopy (EM) - GLP compliance:
- not specified
- Remarks:
- The GLP status was not specified in the article.
- Specific details on test material used for the study:
- - Name of test material: Nanometric cerium dioxide (citrate-coated nano-CeO2)
- Supplier: None (in-house synthesis using a hydrothermal approach)
- Substance type: Monoconstituent substance
- Substance form: Nanoparticulate substance / crystalline nanomaterial in suspension
- Primary particle size (HRTEM, STEM, XRD): Average diameter of 31.2 ± 17.1 nm
- Particle size distribution (HRTEM, STEM, XRD, DLS): Bimodal distribution when suspended in water, with 41 nm (100% of the number and 36% of the volume of nano-CeO2) and 273 nm (64% of the remaining volume)
- Stability: Agglomeration/aggregation tendency in aqueous medium according to particle size distribution and TEM observations
- Specific surface area (BET): 15 m²/g corresponding to an average diameter of 52 nm
- Surface charge (electrophoretic mobility method): Zeta potential of -56 ± 8 mV in water at pH ~7.3
- Isoelectric point: No data available
- Shape (HRTEM, STEM, XRD): Cubic
- Crystallinity (HRTEM, STEM, XRD): Face-centred cubic
- Analytical purity / impurities (ICP-MS): Mentioned as highly pure (99.1% after autoclaving)
- Number density of nano-CeO2 in the suspension: No data available
- Cerium content in nano-CeO2 suspension (ICP-MS): 5.2 ± 0.1% (Yokel RA et al., 2012); ca. 5% (Hardas SS et al., 2014)
- Solubility: No data available
- Oxidation degree (EELS): Enrichment of Ce(III) at the particle rim (surface layer)
- Surface properties (TGA): ca. 18% surface citrate coating
- Lot/batch No.: No data available
- Expiration date of the lot/batch: No data available
- Other information: The nano-CeO2 suspension had a pH of 3.9; free Ce content <<1% after washing
Further explanations on the physico-chemical characterisation of CeO2 nanoparticles are presented below in "any other information on materials and methods incl. tables". - Radiolabelling:
- no
- Species:
- rat
- Strain:
- Sprague-Dawley
- Sex:
- male
- Details on test animals or test system and environmental conditions:
- TEST ANIMALS
- Source: Harlan (Indianapolis, USA)
- Age at study initiation: ca. 70 days old (Hardas SS et al., 2014)
- Weight at study initiation: 300 ± 19 g (Yokel RA et al., 2012); 323 ± 36 g (Hardas SS et al., 2014)
- Fasting period before study: No data available
- Housing: Individually
- Individual metabolism cages: Yes (Yokel RA et al., 2012)
- Diet: Ad libitum (2018 Harlan diet)
- Water: Ad libitum (reverse osmosis [RO] water)
- Acclimation period: No data available
ENVIRONMENTAL CONDITIONS
- Temperature: 21.1 ± 13.3°C
- Humidity: 30-70%
- Air changes: No data available
- Photoperiod: 12 hrs dark / 12 hrs light
IN-LIFE DATES: No data available - Route of administration:
- intravenous
- Vehicle:
- water
- Details on exposure:
- Rats were prepared for i.v. nano-CeO2 (or water, the vehicle) and 1.8% sodium chloride (saline) infusion by surgical implantation of cannula into each femoral vein, that terminated in the vena cava. A day or two later the nano-CeO2 (or vehicle) were infused over 1 h.
- Duration and frequency of treatment / exposure:
- A single exposure (sacrifice 1 and 20 hours, and 1, 7, 30 and 90 days after i.v. infusion)
- Dose / conc.:
- 0 mg/kg bw (total dose)
- Remarks:
- (control injected with water adjusted to pH 3.9 according to Yokel RA et al. (2012))
- Dose / conc.:
- 100 mg/kg bw (total dose)
- Remarks:
- basis: nominal nano-CeO2 dose (as ca. 5 % aqueous citrate-stabilized CeO2 suspension)
- Dose / conc.:
- 87 mg/kg bw (total dose)
- Remarks:
- basis: analytical nano-CeO2 dose (as ca. 5 % aqueous citrate-stabilized CeO2 suspension)
- No. of animals per sex per dose / concentration:
- - Yokel RA et al. (2012)
1-, 7-, and 30-day terminations: 3 per group (control and treated)
90-day termination: 6 (control) or 7 (treated) per group
- Hardas SS et al. (2014)
1- and 20-hour terminations: 5 per group (control and treated)
1- and 7-day terminations: 3 per group (control and treated)
30-day termination: 10 (control) or 11 (treated) per group
90-day termination: 6 (control) or 7 (treated) per group - Control animals:
- yes, concurrent vehicle
- Details on study design:
- All rats were cage-side observed daily to assess respiratory, musculo-nervous, ophthalmic, and other abnormalities (the presence of dyspnoea, tachypnea, tremor, ataxia, head tilt, hyperactivity, hypoactivity, lethargy, paralysis, opacity of the eyes, dilated/constricted pupils, exophthalmia, enophthalmia, conjunctivitis, abnormal secretions/crusting of the eyes, corneal ulcers, diarrhoea, ruffled fur, urine staining, and other notable observations). Each was weighed weekly. Rats scheduled for termination at 7, 30, or 90 days were housed in metabolic cages from 3 days before until the 7th or 14th day after infusion. Urine and faeces were collected daily from 6 control and 8 CeO2–treated rats to 7 days and 6 control and 5 CeO2–treated rats to 14 days (Yokel RA et al., 2012).
- Details on dosing and sampling:
- PHARMACOKINETIC STUDY (Absorption, distribution, excretion)
- Yokel RA et al. (2012)
* Cerium (Ce) content in tissue was determined by ICP-MS and electron microscopy (EM). The method detection limit (MDL) of Ce was of 0.089 mg Ce/kg in tissue and 0.018 mg Ce/L in blood and serum samples. Cerium concentrations below MDL were reported as 50% of the MDL.
* Tissues and body fluids sampled: Urine, faeces, brain cortex, lung, thymus, heart (horizontal medial cross-section), spleen (medial tip), liver (near tip of the larger of the two bifurcated lobes), adrenal, right kidney (medial cross-section), intestine (a 1 cm segment 5–6 cm below the pyloric sphincter), testicles (internal issue), skeletal muscle from the right femur, skin (from the middle of the back), bone marrow (tibia), whole blood (vena cava), cerebrospinal fluid (CSF) (withdrawn from the cisternomedullary space), and skeletal system (cranium, spinal column, ileac crest [pelvis], femur).
Organ/system Ce concentration in all tissues/fluids as well as mass amount of Ce and percentage of the CeO2 dose in the rat were calculated, the latter from the organ weight or based on 0.02, 1.8, 50, 20, 3, and 7% of the body weight for the adrenal gland, intestine, skeletal muscle, skin, bone marrow, and blood, respectively. The skeletal system was separated into four components (cranium, spinal column, pelvis, and femur), which constituted 21, 38, 10, and 31% of its weight, respectively.
* Time and frequency of sampling: Daily for urine and faeces; at termination for other tissues (1, 7, 30, or 90 days post-infusion)
* The rat chow consumed was also analysed by ICP-MS
- Hardas SS et al. (2014)
To determine Ce levels, brain, liver and venous blood samples were collected when the rats were terminated. Ce content in tissue (i.e., liver and brain) and blood samples were measured by ICP-MS. Terbium was added in diluted samples as an internal standard and analysed compared to Ce standards. Analysis of duplicate samples and a duplicate sample spiked with a known amount of Ce included with every 20 samples were concurrently processed. The Ce concentration in blanks and 480% of the samples from the control rats was below the instrument detection limit (0.02–0.03 mg/L).
OTHER
- Yokel RA et al. (2012)
* HISTOLOGICAL EVALUATION:
After termination of ketamine-anaesthetized rats, harvested tissue samples were immediately immersed in 10% neutral buffered formalin and subsequently processed by paraffin embedding for histopathology analysis. Sections (5 µm) were cut and stained with hematoxylin and eosin (H&E) and examined for qualitative and quantitative changes. For plastic embedding, tissues were cut into 3 mm3 pieces, post-fixed in osmium tetraoxide, dehydrated, and embedded in Araldite 502. One-micron sections were cut and stained with toluidine blue. All sections were coded and examined by an experienced histologist and an experimental pathologist.
* OXIDATIVE STRESS ASSESSMENT:
Protein carbonyl (PC) levels, as a global marker for protein oxidation and oxidative stress, were measured in liver and spleen using a specific antibody and slot blot technique. The liver and spleen were examined because they had the highest Ce concentrations. - Statistics:
- Grubb’s test was used to identify outliers in fluid, tissue, urine, faeces Ce results.
A two-way ANOVA was used to compare body weights of rats terminated 90 days after treatment, normalised to their treatment day weight. Organ weights, not and normalised to body weight, were compared by one-way ANOVAs followed by Tukey’s multiple comparison tests and unpaired t-tests. The percentage of the CeO2 dose in the liver, spleen, kidney, heart, lung, brain, thymus, and testicles was calculated based on each rat’s whole organ weight times its Ce concentration, correcting for Ce as a percentage of CeO2. Median pre-treatment 24 h-urine and faeces Ce excretion obtained from 24 rats was subtracted from post-treatment values of CeO2-treated rats to obtain the excretion of Ce attributable to treatments.
The concentration of Ce and percentage of dose in all sampled tissues and fluids and of the four skeletal system regions were compared by two-way ANOVAs and Bonferroni multiple comparisons.
One-way ANOVAs with Tukey tests were also conducted to determine differences in the percentage of dose across time in each sampled tissue or fluid and across tissues and fluids at the same time.
Results were reported as mean ± SD. Significance was accepted at p < 0.05. - Preliminary studies:
- No data available
- Details on absorption:
- Yokel RA et al. demonstrated that after a single exposure CeO2 was detected in blood from day 1. The concentration decreased at days 7 and 30, but increased at day 90. Hardas SS et al. further showed that the tested nano-CeO2 was rapidly cleared from circulating blood (see in Table 2 below). Blood cerium rose sharply in the rats terminated 90 days after nano-CeO2 administration (3.81 ± 2.67% of the nano-CeO2 dose). According to Hardas SS et al., this slight increase observed in blood may be due to the partial dissolution of nano-CeO2 observed in the liver at that time (see Graham UM et al., 2014).
- Details on distribution in tissues:
- - Yokel RA et al. (2012)
CeO2, as Ce, was detectible in all tissues and fluids sampled at 90 days. There was no appreciable clearance of nano-CeO2 over the 90 days, which was mostly present as intracellular CeO2 agglomerations in mononuclear phagocyte system tissues. Of the 64% of nano-CeO2 dose found in the sampled organs and fluid compartments 90 days after its administration, 72% was in the liver, spleen, and bone marrow.
A significant CeO2 decrease was found in the spleen from day 1 compared to days 7 and 30 followed by a significant increase from days 7 and 30 compared to day 90.
Brain Ce concentration, corrected from the cerium in the blood vessels of the brain, in concert with extensive light and electron microscopic observations, suggested very little nano-CeO2 crossed the blood-brain barrier into brain cells.One-way ANOVAs with Tukey tests of the percentage of the dose in each sampled site over time were conducted. They revealed significant reductions in heart (from day 1 to days 7, 30 and 90) and brain (from day 1 to day 90).
When all sample sites were compared at a single time (1, 7, 30 or 90 days), the accumulation in liver was higher than in all other sites but bone marrow. Bone marrow was higher than all other sites at day 1; liver and bone marrow were higher than all other sites at day 7; liver was higher than all sites except bone marrow at day 30; liver was higher than all sites but spleen, spleen was higher than all sites but bone marrow, and bone marrow was higher than all other sites but blood at day 90.
These results showed a little decrease of Ce in the sampled sites over time; much more CeO2 in the liver, bone marrow, and spleen than other sites; and evidence of translocation to the spleen at day 90.
Nano-CeO2 was also distributed in the four skeletal system regions: the spinal column and pelvis had considerably higher Ce concentration than the femur and cranium.
The mean rat chow Ce concentration was 0.22 mg Ce/kg. Given that Sprague Dawley rats eat ca. 5 g food/100 g body weight daily, the daily Ce intake from food of these rats would be ca. 4.4 µg, and over 90 days 0.3 mg, or less than 2% of the Ce in the 87 mg CeO2/kg i.v. dose. Cerium is poorly absorbed from the gastrointestinal tract, as is nano-CeO2. Therefore, Ce in the rat chow did not significantly contribute to blood and tissue Ce levels.
The histological evaluation revealed that liver, spleen, and bone marrow had the most avid uptake and retention of nano-CeO2.
The CeO2-containing Kupffer cells situated in the sinusoids became visible by light microscopy 1 day after infusion. Less visible was hepatocellular accumulation. The continued presence of CeO2 after the single vascular infusion was associated with hepatic granuloma formation first seen after 30 days that persisted in the 90-day samples in all CeO2 infused rats. These intrasinusoidal cellular agglomerates comprised a core of Kupffer cells surrounded by mononucleated cells.
In spite of the granulomatous formation, hepatic parenchyma remained viable. No progression or regression of the granulomatous changes in the liver was seen over the 90 days. Occasional perivascular accumulation of lymphocytes was also observed, and one T-cell population in the granuloma was identified by CD3 immunohistochemistry.
In the enlarged spleen, histological examination showed CeO2-containing macrophages both in the red and white pulp in 30- and 90-day samples. The spleen of many CeO2-treated rats showed punctate white specs.In the brain, light microscopy did not reveal cells with CeO2 accumulation in neurites or the microvessels in the hippocampus or the cerebellum.
- Hardas SS et al. (2014)
Very little CeO2 was in the brain (< 0.018 ± 0.016% of the nano-CeO2 dose), much of which could be accounted for by the blood within the vessels perfusing the brain (see in Table 2 below). Ce was mainly found in the liver with 21 to 40% of the nano-CeO2 dose accumulated in the reticulo-endothelial organ. The % of nano-CeO2 accumulated in the liver decreased over time being of 40 ± 7% at 1 h post-infusion vs. 21 ± 8% at 90 days post-infusion. - Details on excretion:
- Yokel RA et al. observed that there was no significant difference in daily wet faeces outputs during the first week between CeO2-treated and control rats. The only significant differences in urine outputs were greater output on day 3 among the CeO2-treated rats terminated at 1 week compared with many of the other groups and a lower output on day 4 compared with some groups.
Baseline (pre-CeO2 treatment) 24 h urinary and faecal Ce excretion medians were 0.038 and 1.04 µg, respectively. When pre-treatment 24 h median Ce excretion was subtracted from total Ce excretion post CeO2 dosing, ca. 2 and ca. 100 µg of CeO2 appeared in the urine and faeces in the first 2 weeks, representing ca. 0.01 and ca. 0.5% of the dose, respectively.
Hardas SS et al. also mentioned that Ce excretion was of very low rate (unpublished findings). - Metabolites identified:
- not measured
- Bioaccessibility (or Bioavailability) testing results:
- No data available
- Conclusions:
- A single nano-CeO2 administration initially reduced body weight gain, but nor morbidity or mortality, neither profound toxicity were observed. Cerium excretion following CeO2 infusion was 98% in the faeces and very slow, resulting in elimination of < 1% of the dose in the first 2 weeks. Spleen weight was greater at most times after CeO2 administration, associated with visual and microscopic evidence of abnormality. Nano-CeO2 were retained in mononuclear phagocyte system tissues (i.e., liver, spleen) from which there was little clearance over 90 days, supporting thus a significant amount of nano-CeO2 might persisted in rats beyond 30 days. Little or no nano-CeO2 accumulated in the brain. However, significant oxidative responses were observed in the hippocampus of rats i.v. exposed to nano-CeO2, with a maximum response 30 days after infusion; nevertheless a total recovery was observed 90 days after infusion.
The lack of morbidity and mortality, associated with the lack of significant progression (or regression) of adverse changes in the liver and spleen, and the total regression of oxidative changes in the brain failed to support the hypothesis of progression of untoward effects beyond 30 days.
=> Interpretation of results: bioaccumulation potential in reticulo-endothelial organs - Executive summary:
Yokel RA et al. (2012) ascertained the distribution, translocation, elimination and selected biological effects (including histopathology and oxidative stress) in rats after a single intravenous (i.v.) administration of nanometric cerium dioxide (nano-CeO2). In a second study from the same research team, Hardas SS et al. (2014) aimed at characterising the biodistribution of the same nano-CeO2 from blood into brain up to 90 days after systemic administration.
A water suspension of 5% crystalline nano-CeO2 was used in this study. The in-house synthesised nano-CeO2 had a primary particle size of 31 nm, a cubic shape, a crystalline structure, a weak surface area of 15 m²/g and a Ce3+ surface enrichment. Although the particles were coated with citrate stabiliser, the tested nano-CeO2 showed a slight agglomeration/aggregation tendency in aqueous media. The zeta potential of nano-CeO2 was -56 mV, at pH 7.3. In addition, no impurity was detected in the nano-CeO2 suspension.
Male Sprague Dawley rats (3 to 11/group) were i.v. infused water (vehicle control) or a nano-CeO2 suspension at an analytical dose of 87 mg/kg. Rats were then terminated 1 and 20 hours, and 1, 7, 30, or 90 days post-exposure. Cage-side observations were obtained daily and body weight weekly. Daily urinary and faecal cerium (Ce) outputs were quantified for 2 weeks. Nine organs were weighed and samples collected from 14 tissues/organs/systems, blood and cerebrospinal fluid for Ce determination. A histological analysis was performed using electron microscopy. CeO2 concentration and chemical speciation in the brain and/or liver were assessed by inductively coupled plasma mass spectrometry (ICP-MS) and electron energy loss spectroscopy (EELS). Oxidative stress was assessed in liver and spleen by measuring protein carbonyl (PC) levels.
No mortality and no adverse effects were seen after administration of 87 mg CeO2/kg to rats.
Yokel RA et al. demonstrated that less than 1% of the administered nano-CeO2 was excreted in the first 2 weeks, with 98% of eliminated CeO2 found in faeces. Body weight gain was initially impaired. Spleen weight was significantly increased in some CeO2-treated groups, associated with abnormalities. CeO2 was primarily retained in the spleen, liver, and bone marrow. There was no relevant decrease of CeO2 in any tissue over the 90 days. Granulomas were observed in the liver. Time-dependent oxidative stress changes were seen in the liver and spleen. Indeed, increased PC levels in liver were found at most time points (1, 7, and up to 30 days). In contrast, PC levels were decreased in the spleen at each time point examined. It cannot be excluded that these oxidative responses might have resulted from the acidic pH of nano-CeO2 suspension (i.e., 3.9). After 90 days nano-CeO2 induced anti-oxidant effects in both organs. In addition, nano-CeO2 was persistently retained by organs of the mononuclear phagocyte system.
Hardas SS et al. further showed that even after a long-term retention time of 90 days in the liver nano-CeO2 continued to display a significant +3 valence on the surface. Nano-CeO2 was rarely found in the brain.
In conclusion, the tested nano-CeO2 remained in the mammal model, mainly in reticulo-endothelial organs (i.e., liver and spleen), up to 90 days after its i.v. administration. Moreover, the particles exerted pro-oxidant effects in liver and spleen, as well as in the brain with a maximum response at 30 days post-infusion. After 90 days an antioxidant response was induced in the reticulo-endothelial organs. Hardas SS et al. mentioned that some dissolution of nano-CeO2 occurred in the liver 90 days after its i.v. administration (Graham UM et al., 2014), that presumably released Ce ion; some of these ions could have resulted in formation of ultra-small nano-CeO2 in the liver that inherently had, due to their small size, a greater percentage of Ce3+ on their surface, the valence state that enables the antioxidant effects of nano-CeO2 (Graham UM et al., 2014), thus inducing the recovery observed at 90 days post-infusion. The results obtained in both studies suggested that the oxidative effects observed could be an adaptive response of rat organism (i.e., hormesis) as mentioned by Hardas SS et al.
- Endpoint:
- basic toxicokinetics in vivo
- Type of information:
- experimental study
- Adequacy of study:
- weight of evidence
- Study period:
- Not applicable
- Reliability:
- 2 (reliable with restrictions)
- Rationale for reliability incl. deficiencies:
- study well documented, meets generally accepted scientific principles, acceptable for assessment
- Remarks:
- ; but test not conducted under GLP conditions.
- Reason / purpose for cross-reference:
- reference to other study
- Reason / purpose for cross-reference:
- reference to other study
- Objective of study:
- other: hepatic biotransformation
- Qualifier:
- no guideline followed
- Principles of method if other than guideline:
- - Unique intravenous (i.v.) injection of a suspension of nanometric cerium dioxide (nano-CeO2) in rats; sacrifice of animals 1 and 20 hours, and 30 or 90 days after injection
- Biotransformation in liver: Light microscopy (LM), transmission electron microscopy (TEM), scanning transmission electron microscopy (STEM), electron energy loss spectroscopy (EELS) , high-resolution transmission electron microscopy (HRTEM) - GLP compliance:
- not specified
- Remarks:
- The GLP status was not specified in this article.
- Radiolabelling:
- no
- Species:
- rat
- Strain:
- Sprague-Dawley
- Sex:
- male
- Details on test animals or test system and environmental conditions:
- TEST ANIMALS
- Source: No data available
- Age at study initiation: Described as adult
- Weight at study initiation: 316 ± 27g
- Fasting period before study, housing, individual metabolism cages: No data available
- Diet: Ad libitum (2018 Harlan diet)
- Water: Ad libitum (reverse osmosis water)
- Acclimation period: No data available
ENVIRONMENTAL CONDITIONS
- Temperature: 21°C
- Humidity: 30-70%
- Air changes: No data available
- Photoperiod: 12 hrs dark / 12 hrs light
IN-LIFE DATES: No data available - Route of administration:
- intravenous
- Vehicle:
- water
- Remarks:
- adjusted to pH 3.9
- Details on exposure:
- A cannula was surgically inserted, under sterile conditions, into each of the femoral veins and extended into the vena cava. The following day, non-anaesthetised rats were intravenously (i.v.) infused via the shorter cannula with a nano-CeO2 suspension or water (controls) adjusted to pH 3.9 with citric acid, concurrently with an equal volume and rate of 1.8% saline infused into the second cannula to achieve net infusion of iso-isomotic solution. Each fluid was i.v.-infused at rates of 2 mL/kg/h over a duration of 1 h. The cannulas were surgically removed from 30- and 90-day rats.
- Duration and frequency of treatment / exposure:
- A single exposure (sacrifice 1 h, 20 h, 30 days or 90 days after i.v. infusion)
- Dose / conc.:
- 0 mg/kg bw (total dose)
- Remarks:
- (control)
- Dose / conc.:
- 85 mg/kg bw (total dose)
- Remarks:
- basis: analytical nano-CeO2 dose
- No. of animals per sex per dose / concentration:
- Control rats terminated at:
* 1 h: n = 5
* 20 h: n = 8
* 30 days: n = 10
* 90 days: n = 6
Exposed rats terminated at:
* 1 h: n = 5
* 20 h: n = 5
* 30 days: n = 14
* 90 days: n = 7 - Control animals:
- yes, concurrent vehicle
- Details on study design:
- - Dose selection rationale: The high dose was mainly used because of a previous study that was centred on characterising the safety and toxicity of nano-CeO2 (Yokel RA et al., 2012 and 2013).
- Rationale for animal assignment: No data available - Details on dosing and sampling:
- CHARACTERISATION OF HEPATIC NANO-CeO2 CONTENT
Characterisation of nano-CeO2 in liver tissue was done using light microscopy and also using transmission electron microscopy (TEM).
Light microscopy was used to tabulate size and density of lesions inside liver where nano-CeO2 was located. Thin tissue sections (ca. 3 mm²) were immersion fixed, dehydrated and then embedded. Sections were stained with hematoxylin & eosin (H&E) and screened for nano-CeO2-containing Kupffer cells. Nano-CeO2-induced cellular degeneration and proliferation in liver tissue were determined by TUNEL and proliferating cell nuclear antigen (PCNA) immunostaining.
TEM imaging was performed using thin sections (800 Å) without staining. Scanning transmission electron microscopy (STEM) was used to locate nano-CeO2 in liver tissue. Electron Energy Loss Spectroscopy (EELS) analyses were performed at the nanoscale to study the oxidation degree of Ce as a function of particle size and were done in combination with high resolution TEM (HRTEM). - Statistics:
- No data available
- Preliminary studies:
- No data available
- Details on absorption:
- No data available
- Details on distribution in tissues:
- According to Graham UM et al., spleen and liver had sequestered approximately the same amount of nano-CeO2. The authors mentioned that details on nano-CeO2 distribution, clearance and biopersistence were described in the articles of Yokel RA et al. (2012 and 2013).
- Details on excretion:
- No data available
- Details on metabolites:
- - CHARACTERISATION OF HEPATIC NANO-CeO2:
Nano-CeO2 formed agglomerates in the liver up to day 30, but otherwise showed no indication of breakdown or chemical transformation. However, after 90 days inside the liver, i.v.-infused cube-shaped nano-CeO2 had become highly fragmented and rounded along their edges, which indicated that in vivo processing of the particles had occurred. Moreover, accumulations of copious ultrafine crystallites (1 to 3 nm) that formed in the liver within close proximity to the rounded nano-CeO2 were observed and labelled as CeO2 clouds. These second-generation CeO2 particles (crystallites in CeO2 clouds) seemed to be trapped in "groups or swarms" owing to fast precipitation.
Very large polycrystalline CeO2 (up to hundreds of nm) with rounded, partially missing corners was observed in the liver (not seen in the spleen) after 90 days. Since the as-synthesised nano-CeO2 was dense crystals, the authors suggested that the large polycrystals formed in situ of the liver. Many of the large polycrystalline CeO2 grains had rounded corners suggesting there was post-formation dissolution occurring (high energy edge sites) and in some cases the corners had formed pits or cavities. According to Graham UM et al., this further evidenced that nano-CeO2 in the liver underwent a dynamic transformation and bioprocessing.
The redox potential of nano-CeO2 both after hydrothermal synthesis and after in vivo processing in the liver was assessed with EELS. The core region of both freshly synthesised nano-CeO2 cubes and in vivo processed grains always showed characteristic satellite peaks, which suggested a highly equilibrated content of Ce4+ while surfaces of the same particles were high in Ce3+. Thus, a significant enrichment in Ce3+ was systematically observed in surface layers whereas the core regions were primarily Ce4+; reduction potential of as-synthesised CeO2 cubes and that of processed rounded grains in the liver were essentially the same. However, EELS analyses of ultrafines (1 to 3 nm) from CeO2 clouds indicated a reduced valence state (Ce3+) for the entire grains, with a range from 38 to 70% Ce3+. According to the authors, this indicated that CeO2 cloud formation shifted the overall redox activity of CeO2 to a more reduced state and, thus, higher free-radical scavenging activity.
Much lesser amounts of nano-CeO2 seemed to have been bioprocessed inside the spleen which was evidenced by minor occurrence of ultrafines or CeO2-clouds in the spleen. Also, there was less rounding of the sequestered nano-CeO2 in the spleen suggesting that dissolution of nano-CeO2 was less favoured in the spleen environment compared with the liver. Graham UM et al. suggested that dissolution of nano-CeO2 could be linked to the occurrence of the CeO2-clouds.
According to the authors, rod-shaped crystallites were also formed in the liver near in vivo processed nano-CeO2; they were composed of Ce, O, and P and were of comparable size and morphology as sol-gel-derived Ce phosphates. Since significantly more CeO2 clouds formed in the liver than in the spleen (even though the spleen had sequestered approximately the same amount of nano-CeO2), the liver environment could promote the processing of nano-CeO2 through its localized liver acid-base physiology (cellular pH is tightly regulated and nano-CeO2 would primarily encounter a low pH in lysosomes). The in situ formation of CeO2 clouds therefore could be governed by the release of Ce ions derived from dissolving i.v.-infused CeO2 in the liver since the CeO2 dissolution rate is a function of local pH and electrochemical potential.
Thus, in vivo processing led to a much greater reactive nano-CeO2 surface area in the second-generation CeO2 (CeO2 clouds). EELS of the cloud particles demonstrated that the oxidation degree, when compared with the original nano-CeO2, was more reduced and Graham UM et al. suggested that in vivo processing was a function of 1) the acidic environment, 2) the oxidizing potential of the environment, and 3) the exposure time (it took 90 days before the effects of in vivo processing were recognized in tissue samples).
Graham UM et al. then set out to determine how fast the second-generation nano-CeO2, after exposure to an oxidant would be able to return back to the reduced state (Ce4+ => Ce3+), since EELS demonstrated that all particles in the second-generation CeO2 clouds were in a reduced state. As XPS could not be performed directly on CeO2 clouds because nanoparticles could not be isolated because of strong liver tissue interactions, the authors synthesised nano-CeO2 of 2 to 4 nm to resemble the crystallites in CeO2 clouds. To mimic the redox activity of CeO2 clouds, Graham UM et al. first performed oxidation experiments (using 0.5m H2O2 that quickly evaporated) and oxidized the particles. The 2-4 nm nano-CeO2 (as-synthesised) had approximately 40% Ce3+ before H2O2 exposure and approximately 0% Ce3+ afterwards, and furthermore in less than 1 h transitioned back to the pre-H2O2 baseline composition (i.e., around 40% Ce3+). Thus, oxidized ultrafine CeO2 returned quickly and completely to the reduced state without the presence of reducing agents.
The authors proposed the following predictive model for the in vivo transformation and reducing potential of ultrafine CeO2 in the: 1) nanoparticle sequestration in a complex acid–base-controlled environment (liver) with some dissolution of original particles; 2) in situ formation of second-generation CeO2 (CeO2 clouds); 3) greater reactive surface area for the second-generation CeO2 inside CeO2 clouds; and 4) higher Ce3+ associated with second-generation CeO2. This in vivo transformation of CeO2 could be a dynamic system.
According to the authors, the model established a temporal link between cellular processes in the brain (Hardas SS et al., 2014) and the in vivo transformation of CeO2 in the liver. Prior to in vivo processing of nano-CeO2, oxidative stress gradually increased in CeO2-treated rats and brain cellular stress (stage S1; 1 h-1 d; antioxidant defence) was then followed by brain inflammation (stage S2; 1-7 d; pro-inflammatory response) and then brain-cell death (stage S3; 7-30 days; mitochondrial-mediated cytotoxic effects). However, after 90 days an unexpected brain cellular clearance response was reported (Hardas SS et al., 2014), which corresponded in the model to stage S4 (30-90 days) and indicated a reversal or decrease in oxidative stress. Brain oxidative stress was shown to have increased as early as 1 h after nano-CeO2 infusion (signalling pathway: Nrf-2), and 1 day later triggered brain inflammation (signalling pathway: TNF-α (NF-κB IL-1β)). The model also showed that between 7 and 30 days, inflammation was significantly increased in the hippocampus, cortex, and cerebellum, and brain-cell death occurred at 30 days (signalling pathway: pro-apoptotic; Pro-Caspase-3), and then followed by autophagy (LC-3AB), and finally returned to normal levels at 90 days. Importantly, the late cellular and protein-clearance response previously observed in the rat brain (Hardas SS et al., 2014) coincided with the inception of in vivo processing of nano-CeO2. Since the processed nanoparticles in the liver (second-generation CeO2) were shown to have high Ce3+, they may also have a high affinity for reactive oxygen species and free-radical scavenging activity. Although the mechanisms were not established by the authors, they anticipated that the in vivo processing of nano-CeO2 had far-reaching protective effects, as was observed with the reversal in pro-oxidant brain effects. - Bioaccessibility (or Bioavailability) testing results:
- No data available
- Conclusions:
- Intravenously-infused nano-CeO2, after prolonged residence time in the liver (90 days), underwent in vivo processing. Nano-CeO2 undergoes partial dissolution inside the liver that causes a shift towards smaller particle size and an increased reactive surface area with enhanced Ce3+ activity that leads to greater free-radical scavenging potential of the ultrafines. The authors concluded that the breakdown and redistribution could be a step towards improving ceria biocompatibility in vivo.
=> Interpretation of results: bioaccumulation potential in reticulo-endothelial organs and biotransformation in liver - Executive summary:
Graham UM et al. (2014) investigated the in vivo transformation of nanometric cerium dioxide (nano-CeO2) in the rat liver by evaluating nano-CeO2 chemical and structural stability and solubility once sequestered inside the liver.
A water suspension of 5% crystalline citrate-coated nano-CeO2 was used in this study. The in-house synthesised nano-CeO2 had a primary particle size of ca. 30 nm, a cubic shape, and a crystalline structure. The particles showed no or few agglomeration/aggregation tendency in aqueous suspension due to citrate coating which stabilised the suspended particles at a mean size of 31 ± 4 nm. The nano-CeO2 suspension had a pH of 3.9.
The nano-CeO2 suspension was intravenously (i.v.) infused into Sprague-Dawley male rats at 0 (control), or 85 mg/kg nano-CeO2. Animals were terminated 1 h, 20 h, 30 days and 90 days after infusion. CeO2 distribution in liver, lung, kidney, spleen, heart, thymus, and brain was by high resolution transmission electron microscopy (HRTEM) and inductively coupled plasma mass spectrometry (ICP-MS) (for details, see the publications of Yokel RA et al., 2012 and 2013). In addition, the authors characterised the nano-CeO2 content in liver using transmission electron microscopy (TEM), scanning transmission electron microscopy (STEM), electron energy loss spectroscopy (EELS) and HRTEM.
According to Graham UM et al., spleen and liver had sequestered approximately the same amount of nano-CeO2. The general cytoarchitecture of liver tissue was not altered by the sequestered nano-CeO2, but Kupffer cells contained many nano-CeO2 which formed small agglomerates and underwent fusion with lysosomes to form phagolysosomes. Moreover, Kupffer cells with mononucleated lymphocytes had formed large granulomata in the tissue parenchyma, while surrounding hepatocytes rarely showed cytoplasmic inclusion of CeO2 agglomerates.
Nano-CeO2 formed agglomerates in the liver up to day 30, with no indication of breakdown or chemical transformation. However, after 90 days inside the liver, the i.v.-infused cube-shaped nano-CeO2 had become highly fragmented and rounded along their edges, which indicated that in vivo processing of the particles had occurred. Moreover, accumulations of copious ultrafine crystallites (1 to 3 nm) that formed in the liver within close proximity to the rounded nano-CeO2 were observed and labelled as CeO2 clouds. This represented a second generation of CeO2. EELS of the cloud particles demonstrated that the oxidation degree, when compared with the original nano-CeO2, was more reduced (38 to 70% Ce3+). This measurable change in the valence reduction of the second-generation CeO2 could be linked to an increased free-radical scavenging potential.
Much lesser amounts of nano-CeO2 seemed to have been bioprocessed inside the spleen.
In conclusion, i.v.-infused nano-CeO2, after prolonged residence time in the liver (90 days), underwent in vivo processing. Nano-CeO2 undergoes partial dissolution inside the liver that causes a shift towards smaller particle size and an increased reactive surface area with enhanced Ce3+ activity that leads to greater free-radical scavenging potential of the ultrafines. The authors concluded that the breakdown and redistribution could be a step towards improving CeO2 biocompatibility in vivo.
- Endpoint:
- basic toxicokinetics in vivo
- Type of information:
- experimental study
- Adequacy of study:
- weight of evidence
- Study period:
- Not applicable
- Reliability:
- 2 (reliable with restrictions)
- Rationale for reliability incl. deficiencies:
- study well documented, meets generally accepted scientific principles, acceptable for assessment
- Remarks:
- ; but test not conducted under GLP conditions.
- Reason / purpose for cross-reference:
- reference to other study
- Reason / purpose for cross-reference:
- reference to other study
- Reason / purpose for cross-reference:
- reference to other study
- Reason / purpose for cross-reference:
- reference to other study
- Reason / purpose for cross-reference:
- reference to other study
- Objective of study:
- distribution
- other: blood-brain barrier integrity; biopersistence in brain, liver, and spleen; histopathology; comparison between sizes and shapes of nano-CeO2
- Qualifier:
- no guideline followed
- Principles of method if other than guideline:
- - Unique intravenous (i.v.) injection of a suspension of nanometric cerium dioxide (nano-CeO2) with various physico-chemical properties; sacrifice of animals 1, 20, or 720 hours (30 days) after exposure
- Blood-brain barrier (BBB) integrity: evaluation using fluorescein and horseradish peroxidase (HRP) as markers of BBB permeability
- Nano-CeO2 tissue distribution: quantification using light (LM) and electron microscopy (EM), and inductively coupled plasma-mass spectrometry (ICP-MS)
- Histopathological analysis performed using LM - GLP compliance:
- not specified
- Remarks:
- The GLP status was not specified in this article.
- Radiolabelling:
- no
- Species:
- rat
- Strain:
- Sprague-Dawley
- Sex:
- male
- Details on test animals or test system and environmental conditions:
- TEST ANIMALS
- Source: Harlan (USA)
- Age at study initiation: ca. 75 days old
- Weight at study initiation: 333 ± 35 g
- Fasting period before study: No data available
- Housing: Individually
- Individual metabolism cages: Yes
- Diet: Ad libitum, 2018 Harlan diet
- Water: Ad libitum, reverse osmosis water
- Acclimation period: No data available
ENVIRONMENTAL CONDITIONS
- Temperature: 21.1 ± 13.3°C
- Humidity: 30 to 70%
- Air changes: No data available
- Photoperiod: 12 hrs dark / 12 hrs light
IN-LIFE DATES: No data available - Route of administration:
- intravenous
- Vehicle:
- water
- Details on exposure:
- Each type of nano-CeO2 was prepared as a ~5% suspension in water. At pH below 7.0, 5-nm nano-CeO2 precipitated; therefore it was maintained as an aqueous suspension at pH 7.7 to 8. Each nano-CeO2 was i.v. infused without any further treatment other than the larger nano-CeO2 (i.e., 55 nm) that required agitation during infusion to maintain the dispersion.
Rats were prepared for i.v. CeO2 (or the vehicle water) and 1.8% sodium chloride infusion by surgical implantation of a cannula into each femoral vein, under anaesthesia. The cannulas terminated in the vena cava, and were connected to an infusion pump via a flow-through swivel. This enabled administration of CeO2 particles and observation of its effects in the awake, mobile rat. The day after cannula implantation the non-anaesthetized rat was infused, over 1 h, via the longer cannula with the nano-CeO2 suspension in water, or water. - Duration and frequency of treatment / exposure:
- A single exposure (sacrifice 1, 20, or 720 hours after i.v. infusion)
- Dose / conc.:
- 175 mg/kg bw (total dose)
- Remarks:
- for Ce5 in the preliminary study
basis: nominal nano-CeO2 dose - Dose / conc.:
- 250 mg/kg bw (total dose)
- Remarks:
- for Ce5 in the preliminary study
basis: nominal nano-CeO2 dose - Dose / conc.:
- 100 mg/kg bw (total dose)
- Remarks:
- for Ce30 in the preliminary study
basis: nominal nano-CeO2 dose - Dose / conc.:
- 78 mg/kg bw (total dose)
- Remarks:
- for Ce55 in the preliminary study
basis: nominal nano-CeO2 dose - Dose / conc.:
- 100 mg/kg bw (total dose)
- Remarks:
- for Ce55 in the preliminary study
basis: nominal nano-CeO2 dose - Dose / conc.:
- 250 mg/kg bw (total dose)
- Remarks:
- for Ce55 in the preliminary study
basis: nominal nano-CeO2 dose - Dose / conc.:
- 0 mg/kg bw (total dose)
- Remarks:
- (control)
in the main study - Dose / conc.:
- 100 mg/kg bw (total dose)
- Remarks:
- for Ce5, Ce15 and Ce30 in the main study
basis: nominal nano-CeO2 dose
This corresponded to an analytical dose of 85 mg/kg for Ce5 and Ce30, and 70 mg/kg for Ce15. - Dose / conc.:
- 50 mg/kg bw (total dose)
- Remarks:
- for Ce55 in the main study
basis: nominal nano-CeO2 dose
This corresponded to an analytical dose of 50 mg/kg. - No. of animals per sex per dose / concentration:
- - Ce5:
* 7 controls and 11 treated rats, sacrificed at 1 h post-infusion
* 8 controls and 12 treated rats, sacrificed at 20 h post-infusion
* 7 controls and 7 treated rats, sacrificed at 720 h post-infusion
- Ce15:
* 3 treated rats, sacrificed at 1 h post-infusion
* 5 controls and 5 treated rats, sacrificed at 720 h post-infusion
- Ce30:
* 5 controls and 5 treated rats, sacrificed at 1 h post-infusion
* 5 controls and 5 treated rats, sacrificed at 20 h post-infusion
* 7 controls and 8 treated rats, sacrificed at 720 h post-infusion
- Ce55:
* 5 controls and 5 treated rats, sacrificed at 1 h post-infusion
* 5 controls and 9 treated rats, sacrificed at 20 h post-infusion - Control animals:
- yes, concurrent vehicle
- Details on study design:
- A pilot study was conducted with each of the tested nano-CeO2 to determine the tolerability following i.v. infusion. Rats were infused approximately 250 or 175 mg/kg of Ce5; three of eight died. A dose of approximately 250 mg of Ce15/kg was tolerated. Infusion of 100 mg/kg of the Ce30 resulted in some evidence of mild distress (tachypnea, skittish behaviour and not resting well). Dyspnoea and lethargy were seen in rats given 78, 100, or 250 mg of Ce55/kg. Therefore, the target doses were 100 mg/kg for Ce5, Ce15, Ce30 and 50 mg/kg for Ce55, infused over 1 h.
- Details on dosing and sampling:
- PHARMACOKINETIC STUDY (Absorption, distribution, excretion)
The brain, spleen, liver, right kidney, heart, and lung were weighed. Samples of blood (from a venous line for rats terminated 1 or 20 h after infusion or from trunk blood when decapitated 30 days after infusion), brain cortex, spleen medial tip), and liver (near tip of the larger of the two bifurcated lobes) were collected, weighed, and stored at -20°C for later determination of Ce concentration.
Hippocampus, cerebellum, spleen, liver, and kidney samples were collected for LM and EM histopathology and CeO2 distribution assessment Cerium (Ce) content was also determined by ICP-MS. The Ce method detection limit (MDL) was of 0.089 mg/kg in tissue and of 0.018 mg/kg in blood. The percentage of the nano-CeO2 dose in the brain, liver, and spleen was calculated as: (cerium concentration x organ weight)/CeO2 dose, the latter was based on cerium quantification in the dosing dispersion (determined by ICP-MS) x the dose volume.
The percentage of the CeO2 dose in blood was based on a vascular volume of 7% of the rat's body weight.
OTHER
- BBB INTEGRITY ASSESSMENT:
Rats terminated 1 or 20 h after CeO2 or vehicle infusion were anaesthetized and given i.v. in saline 6 mg sodium fluorescein and 20 mg horseradish peroxidase (HRP) 5 min before termination (3.33 mL/kg). These are small and large BBB permeability markers, respectively. All rats were given diphenhydramine (5 mg/kg) through intraperitoneal route 30 min before the fluorescein-HRP infusion, to inhibit HRP-induced arterial hypotension.
Post-mortem brain samples (ca. 0.25 g) were obtained to quantify fluorescein and HRP.
The brain samples were homogenised by probe sonication in phosphate buffer. The homogenates were then centrifuged, 150 µL of the supernatant was set aside for the HRP assay; the remaining supernatant was accurately weighed for the fluorescein assay. Fluorescein quantification was performed using a fluorimetrer. The HRP activity was determined at 460 nm using a UV spectrophotometer. - Statistics:
- Ce concentrations below the method detection limit (MDL) were assigned 50% of the MDL and included in the statistical analysis as such.
Outliers in blood and tissue Ce concentration results identified by the Grubb's test were not used in data analysis.
Statistical comparison of % of nano-CeO2 dose in blood, brain, liver, and spleen of the control rats for each of the 4 treatment groups was conducted using one-way ANOVA followed by Tukey's post-hoc test and found to not be significant. Results of all control rats for blood and each organ were merged and compared to the % of nano-CeO2 dose in treated rats by Dunnett's test; and within blood or an organ across termination times for each of the four nano-CeO2 by ANOVA with Tukey's post-hoc test or two-tailed unpaired t tests.
Comparison of the % of nano-CeO2 dose in blood or an organ at the same time following infusion of the tested nano-CeO2, as well as the differences in pharmacokinetic parameters was conducted using one-way ANOVAs followed by Tukey's post-hoc tests.
Results from control and treated rats were compared for BBB marker concentration by one-tailed unpaired t tests.
Significance was accepted at p < 0.05, except the mass amount of Ce in the blood or organs as a percentage of CeO2 dose, for which α was adjusted to 0.05/10 to correct for the multiple comparisons.
Results were reported as mean ± standard deviation (SD). - Preliminary studies:
- Three of 8 rats that received Ce5 died. There was evidence of mild distress in rats that received Ce30 (tachypnea, skittish, not resting well) and significant toxicity in rats that received Ce55 (dyspnoea, lethargic).
Therefore, the target doses were 100 mg/kg (ca. 0.58 mM) for Ce5, Ce15, and Ce30 and 50 mg/kg for Ce55. This large CeO2 exposure was used to enhance the ability to detect Ce in the blood and organs up to the 30 days after the single nano-CeO2 infusion. Yokel RA et al. found that the % of Ce5 dose in the liver was comparable after a single i.v. administration of 11 mg CeO2/kg, 5 administrations of this dose given over consecutive days, and a single administration of 56 mg CeO2/kg (unpublished results).
Given the persistence of nano-CeO2 in the mammal model, it was anticipated that small frequent doses will accumulate so that a single large dose, as used in this study, models the result of this accumulation (e.g., multiple smaller doses). - Details on absorption:
- According to Yokel RA et al., Ce15, Ce30, and Ce55 were rapidly cleared from blood as described above. This was supported by the significantly greater % of Ce5 dose in blood 1 and 20 h after its infusion compared to CeO2 in blood taken after the same elapsed times for rats that received the 3 larger nano-CeO2. The longer persistence of Ce5 in blood may relate to its greater extent of citrate coating and/or its small size avoiding ready recognition by reticulo-endothelial organs.
- Details on distribution in tissues:
- - CeO2 BIODISTRIBUTION:
Of the 216 samples from control rats analysed for Ce, 47 were above the MDL of 0.089 mg/kg and 0.018 mg/L. Ten were outliers by the Grubb’s test. Of the 287 sample from nano-CeO2 treated-rats analysed for Ce, all but 27 were above the MDL; 7 were blood samples from rats 30 days after the 30- or 55-nm nano-CeO2 and 20 were brain samples. Samples having less than the MDL value in nano-CeO2-treated rats reflected the low cerium concentration in blood 30 days after nano-CeO2 administration and in brain at all times.
For all control rats, the mean amount of Ce in blood, brain, liver, and spleen, expressed as a % of the CeO2 dose given to the treated rats, was 0.003, 0.001, 0.0004, and 0.04, respectively. Significant differences of the CeO2 dose in blood or organs were found between times after nano-CeO2 administration (see in Table 2 below). According to the authors, there were no significant decreases of Ce in the liver or spleen over time. However, based on the figures available in the article, a trend towards a decrease in Ce could be observed in almost all tissues and particularly at 30 days post-infusion. To the contrary, the percentage of CeO2 dose in the spleen significantly increased over time after administration of Ce15 and Ce30. The sum of the % of CeO2 dose in the blood and 3 organs sampled accounted for 53-62%, 36-38%, 41-45%, 13-24% of the Ce5, Ce15, Ce30, and Ce55 doses, respectively.
There was a significantly greater % of Ce5 dose in blood 1 and 20 h after infusion than the other 3 CeO2 particles. Brain had a significantly greater % of the Ce5 dose than the Ce30 at 1 h and 30 d after infusion. The rats in this work were not perfused to remove blood from their organs when terminated; therefore some of the brain Ce could be attributed to Ce in the blood in the vessels in the brain. Based on the rat brain vascular volume and cerium concentration in blood determined in this study, the Ce found in the brain of nano-CeO2 treated rats could be accounted for by the Ce in circulating blood only in rats terminated 1 h after dosing with Ce5 and Ce30. This suggested some nano-CeO2 associated with the brain microvascular endothelial cells or astrocyte foot processes, or penetrated beyond those cells into brain parenchyma.
The % of the Ce55 dose seemed to be less in brain, liver, and notably in spleen 20 h after infusion than after the smaller sized nano-CeO2. This was probably due to the accumulation of this largest, least citrate-surface stabilised nano-CeO2 at the site of its delivery into the vena cava.
According to the authors, the spleen contained significantly less of the total dose of the 5-nm than the 15-nm CeO2 at 15 days (data not shown), whereas the liver contained more of the 5- than 30-nm CeO2 at 20 h.
- HISTOPATHOLOGY:
Necropsy observation of rats that received Ce55 revealed a white deposit in the vena cava at the tip of the cannula that infused the nano-CeO2, indicating that a significant amount of CeO2 was deposited there during its infusion, and did not circulate in the blood. This was presumably due to the presence of the larger sized particles in this nano-CeO2 suspension and its lower extent of surface citrate coating (ca. 15%).
LM analysis displayed that many CeO2-treated rats that received Ce5 or Ce30 showed punctuate white specs on the spleen surface 30 days after CeO2 infusion that appeared to be similar to deposits observed in the vena cava at the infusion site and were found to be CeO2 agglomerates. Two peripheral organs rich in reticulo-endothelial cells, the liver and spleen, showed a large retention of nano-CeO2. Intra-sinusoidal cell aggregates were formed by CeO2-containing Kupffer cells and adherent mononucleated cells. Ultrastructural study (EM) demonstrated CeO2 uptake and retention in Kupffer cells with most of the internalized CeO2 as agglomerates. Agglomeration of cytoplasmic nano-CeO2 in the spleen appeared similar to the liver.
Nano-CeO2 was not found within mitochondria or the cell nucleus of Kupffer cells or hepatocytes. Considerable effort to detect nano-CeO2 detection in hippocampus and cerebellum from Ce5- and Ce30-infused rats revealed only very rare electron dense particulates lining the luminal side of brain microvascular endothelial cells, but not localizing in the brain parenchyma. - Details on excretion:
- CeO2 excretion was not measured in this study. However, the authors cited previous publications in which faecal and urinary nano-CeO2 excretion was not seen: e.g., < 0.5% excreted within 2 weeks for Ce30 (Yokel RA et al., 2012).
- Metabolites identified:
- not measured
- Bioaccessibility (or Bioavailability) testing results:
- No data available
- Conclusions:
- Regardless of the size or shape, nano-CeO2 mainly accumulated in reticulo-endothelial organs such as liver and spleen. Moreover, the results suggested that BBB could protect the brain from nano-CeO2 circulating in the blood, that a large i.v. dose of these nanomaterials did not greatly disrupt BBB integrity, and that brain delivery of nano-CeO2 is a challenge.
=> Interpretation of results: bioaccumulation potential in reticulo-endothelial organs (liver and spleen) - Executive summary:
Yokel RA et al. (2013) determined the biodistribution, translocation, and persistence of different size of nanometric cerium dioxide (nano-CeO2) in the brain, blood, liver, and spleen following a single intravenous (i.v.) injection to rats.
Four size-different types of nano-CeO2 were used in this study. The different nanomaterials were physico-chemically characterised:
Parameters
Results
Methods
Ce5
Ce15
Ce30
Ce55
Primary
particle size
Expected value
Measured value
5 nm
4.6 ± 0.1 nm {4.8 nm}
15 nm
12.0 ± 0.2 nm {13.5 nm}
30 nm
31.2 ± 0.5 nm {51 nm}
55 nm
55.0 ± 0.2 nm {59 nm}
-
TEM
Particle size
distribution in water
Number basis
[Volume basis]
7 nm [98%, 7 nm; 2%, 18 nm]
25 nm [57%, 25 nm; 43%, 145 nm]
41 nm [36%, 41 nm; 64%, 273 nm]
No data
TEM, dynamic light scattering (DLS)
Stability
Slight agglomeration/aggregation tendency
Slight agglomeration/aggregation tendency
Slight agglomeration/aggregation tendency
No data
-
Specific surface area
[mean diameter
estimated from
surface area]
121 m²/g [6.5 nm]
71 m²/g [11 nm]
15 m²/g [52 nm]
No data
BET method
Surface charge
in water (zeta potential)
-53 ± 7 mV at pH ~7.35
-57 ± 5 mV at pH ~7.3
-56 ± 8 mV at pH ~7.3
-32 ± 2 mV at pH ~7.3
DLS
Shape
Polyhedral
Polyhedral
Cubic
Polyhedral
TEM
Crystallinity
Crystalline
Crystalline
Crystalline
Crystalline
X-ray diffraction (XRD), TEM
Cerium content
in nano-CeO2 suspension
Analytical dose was 85% of the intended 100 mg/kg nominal dose.
70% of the intended 100 mg/kg nominal dose
85% of the intended 100, 100 mg/kg nominal
103% of the intended 50 mg/kg nominal dose
ICP-MS
Surface properties –
Extent of surface
citrate coating
ca. 40% surface coated with citrate
ca. 27% surface coated with citrate
ca. 18% surface coated with citrate
ca. 15% surface coated with citrate
No data
Free Ce content
in suspensions
11.6 ± 0.3%
<< 1%
<< 1%
<< 1%
ICP-MS
pH of suspensions
Maintained at pH 7.7 to 8
pH 3.5
pH 3.9
pH 7
pH metry
{d} mean diameter estimated from TEM measurements
Male Sprague-Dawley rats (3 to 12/group) were i.v. infused with the different nano-CeO2 (as a 5% suspension in water) at the nominal doses of 0 (control), 50 (Ce55) or 100 mg/kg (Ce5, Ce15, Ce30). Control rats received water, which was nano-CeO2 vehicle. Animals were terminated 1, 20, or 720 h later. Blood, brain, spleen, liver, right kidney, heart, and lung samples were collected at these different time points. Cerium (Ce) concentration was also determined by inductively coupled plasma-mass spectrometry (ICP-MS) in blood, brain, liver, and spleen.
The authors reported that the 15-, 30- and 55-nm nano-CeO2 were more rapidly cleared from blood as compared to the 5-nm nano-CeO2. The longer persistence of the Ce5 in blood may relate to its greater extent of citrate coating and/or to its small size avoiding ready recognition by reticulo-endothelial organs. Of the different compartments/tissues studied, ≥ 98% nano-CeO2 were retained 20 h after its i.v. infusion in the reticulo-endothelial organs liver and spleen, from which there was no significant clearance over 720 h. The liver contained significantly more of the total Ce5 dose than of Ce30 at 20 h. The spleen contained significantly more of the Ce15 than the Ce5 at 30 d, suggesting preferential clearance of smaller particles by the liver component mononuclear phagocyte system.
Further, Yokel RA et al. found that < 0.5% of the Ce30 dose was excreted in the urine and faeces within 2 weeks.
Infusion of a large dose of nano-CeO2 produced modest if not brain-blood barrier (BBB) disruption, as evidenced by non-significantly increased brain fluorescein and horseradish peroxidase, not sufficient to allow appreciable brain CeO2 entry. Electron microscopy revealed only occasional nano-CeO2 beyond the BBB.
These results suggested that BBB could protect the brain from nano-CeO2 circulating in the blood, that a large i.v. dose of these nanomaterials did not greatly disrupt BBB integrity, and that brain delivery of nano-CeO2 is a challenge.
- Endpoint:
- basic toxicokinetics in vivo
- Type of information:
- experimental study
- Adequacy of study:
- weight of evidence
- Study period:
- Not applicable
- Reliability:
- 2 (reliable with restrictions)
- Rationale for reliability incl. deficiencies:
- study well documented, meets generally accepted scientific principles, acceptable for assessment
- Remarks:
- ; but test not conducted under GLP conditions.
- Reason / purpose for cross-reference:
- reference to other study
- Reason / purpose for cross-reference:
- reference to other study
- Reason / purpose for cross-reference:
- reference to other study
- Reason / purpose for cross-reference:
- reference to other study
- Reason / purpose for cross-reference:
- reference to other study
- Reason / purpose for cross-reference:
- reference to other study
- Reason / purpose for cross-reference:
- reference to other study
- Objective of study:
- distribution
- other: comparison between sizes and shapes of nano-CeO2 and dosing schedule
- Qualifier:
- no guideline followed
- Principles of method if other than guideline:
- - Unique and repeated (5) intravenous (i.v.) injections of a suspension of nanometric cerium dioxide (nano-CeO2) with various physico-chemical properties; sacrifice of animals 1 hours to 30, and 90 days after exposure
- Nano-CeO2 tissue distribution: quantification using inductively coupled plasma-mass spectrometry (ICP-MS) - GLP compliance:
- not specified
- Remarks:
- The GLP status was not specified in the article.
- Radiolabelling:
- no
- Species:
- rat
- Strain:
- Sprague-Dawley
- Sex:
- male
- Details on test animals or test system and environmental conditions:
- TEST ANIMALS
- Source: Harlan (USA)
- Age at study initiation: ca. 90 days old
- Weight at study initiation: 386 ± 58 g
- Fasting period before study: No data available
- Housing: Individually prior to study and after cannula removal (a few days after the i.v. infusion) in the University of Kentucky Division of Laboratory Animal Resources facility
- Individual metabolism cages: No data available
- Diet: Ad libitum, 2018 Harlan diet
- Water: Ad libitum, reverse osmosis water
- Acclimation period: No data available
ENVIRONMENTAL CONDITIONS
- Temperature: 21.1 ± 13.3°C
- Humidity: 30 to 70%
- Air changes: No data available
- Photoperiod: 12 hrs dark / 12 hrs light
IN-LIFE DATES: No data available - Route of administration:
- intravenous
- Vehicle:
- water
- Details on exposure:
- The rats were infused when not anaesthetized to enable observation of effects produced without the confounding effects of anaesthesia.
Ce5 and Ce30 were infused in water to avoid agglomeration. A concurrent infusion was given of 1.8% sodium chloride at the same rate in a second i.v. cannula to deliver a net isosmotic load to the rat. All nano-CeO2 dispersions were infused at a rate of 2 mL/kg rat body weight over 1 h, with the exception of the 50 mg/kg dose of nanorods, which was infused over 2.5 h.
The dried (citrate-coated) CeR sample was dispersed in 10% sucrose solution by sonication for 10 min and was infused into the rats. - Duration and frequency of treatment / exposure:
- - Single exposure for Ce5 (sacrifice 30 days after i.v. infusion), Ce30 (sacrifice 1 h, 30 and 90 days after i.v. infusion), CeR (sacrifice 1 h and 30 days after i.v. infusion)
- Repeated exposure for control (vehicle) and Ce5 daily for 5 consecutive days (sacrifice 30 days after i.v. infusion) - Dose / conc.:
- 11 mg/kg bw (total dose)
- Remarks:
- for Ce5, as single and repeated exposures
basis: nominal nano-CeO2 dose - Dose / conc.:
- 6 mg/kg bw (total dose)
- Remarks:
- for Ce30, as single exposure only
basis: nominal nano-CeO2 dose - Dose / conc.:
- 20 mg/kg bw (total dose)
- Remarks:
- for CeR, as single exposure only
basis: nominal nano-CeO2 dose - Dose / conc.:
- 50 mg/kg bw (total dose)
- Remarks:
- for CeR, as single exposure only
basis: nominal nano-CeO2 dose - No. of animals per sex per dose / concentration:
- - Single exposure
* Ce5: n = 5 / controls (vehicle): n = 5
* Ce30: n = 4 to 5 / controls (vehicle): n = 3 to 4
* CeR: n = 5 at 20 mg/kg and 9 at 50 mg/kg / controls (vehicle): n = 5
- Repeated exposure
* Ce5: n = 5 / controls (vehicle): n = 5 - Control animals:
- yes, concurrent vehicle
- Details on study design:
- In initial studies, two non-anaesthetized rats were i.v. infused with around 50 or 100 mg/kg CeR over 2.5 or 5 h. The 50 mg/kg dose was tolerated but the rat that received 100 mg/kg was slow, weak, and not responsive to a cage tap. Therefore, the test dose was 50 mg/kg CeR infused i.v. over 2.5 h.
- Details on dosing and sampling:
- PHARMACOKINETIC STUDY (Absorption, distribution, excretion)
- Tissues and body fluids sampled: Liver, bone marrow, spleen, skeletal system, lung, skeletal muscle, fat, heart, kidney, blood, thymus, brain, mesenteric lymph nodes, adrenal
- Time and frequency of sampling: Samples were harvested on rat termination from the abovementioned organs and blood following procedures described by Yokel RA et al. (2012). The authors added that mesenteric lymph nodes and fat were harvested from some rats, as nano-CeO2 deposition to these sites had not been previously reported to their knowledge.
Samples were prepared and analysed for cerium (Ce) by microwave-assisted nitric acid/hydrogen peroxide digestion and inductively coupled plasma mass spectrometry (ICP-MS), as previously described (Yokel RA et al., 2009). The method detection limits (MDL) were 0.089 mg Ce/kg for tissues and 0.018 mg Ce/L in blood samples. Spike recovery for ICP-MS analyses was 94 ± 3%. Average relative percentage difference between replicate analyses was 3.2 ± 2.0%.
Ce concentrations below the MDL were assigned 50% of the MDL and included in the statistical analysis as such. The percentage of the nano-CeO2 dose in the adrenal and thymus glands, brain, heart, kidney, liver, lung, and spleen was calculated as Ce concentration in the analysed sample multiplied by the weight of the organ, as harvested, divided by nano-CeO2 dose. The nano-CeO2 dose was determined as the Ce concentration in the dosing dispersion (determined by ICP-MS) times the dose volume. The percentage of the nano-CeO2 dose in blood was based on a vascular volume of 7% of the rat's body weight. The percentage of the nano-CeO2 dose in the lymph node (0.4% of body weight) was based on the lymph nodes of the rat, weight of the mesenteric lymph nodes (0.3 gm) and average weight (20 mg) of many other lymph nodes. The percentage of the nano-CeO2 dose in other organs was based on reported values; bone marrow (3%), skeletal muscle (45%), and fat (12.5%). - Statistics:
- Outliers in blood and tissue Ce concentration results identified by the Grubb's test were not used in data analysis.
Results of control rats for blood and each organ were compared to the % of the nano-CeO2 dose in treated rats by an unpaired one-tailed t-test with Welch's correction for unequal variances and alpha < 0.05 adjusted with Bonferroni correction for multiple comparisons. Results of nano-CeO2 treated rats were compared within blood or organs across termination times (for Ce30 and CeR), and/or total dose (for Ce5 and CeR), for each of the groups that received the same nano-CeO2, and across all treatments of this study plus prior studies of the same research group (Yokel RA et al., 2012 and 2013) for liver, spleen and bone marrow by ANOVA with Tukey's post hoc test or two-tailed unpaired t-tests. Results were reported as mean ± S.D.
An analysis of the percentage of the dose in each sampled organ compared to the percentage that would be expected if the tested nano-CeO2 non-selectively distributed, a relative deposition index, was conducted. Organ volumes were averages from the studies of Yokel RA et al. or from studies published by other research's teams for blood, lymph node, bone marrow, skeletal muscle, and fat. By relating the amount in an organ to its size, this calculation identified sites of preferential nano-CeO2 accumulation. - Preliminary studies:
- No data available
- Details on absorption:
- No data available
- Details on distribution in tissues:
- Of the 293 samples from control rats analysed for Ce, 56 (19%) were above the MDL of 0.089 mg/kg for tissue and 0.018 mg/L for blood. These were most commonly in the liver, spleen, brain, bone marrow, bone, and lung. Twenty-three values from control subjects were outliers by the Grubb's test, mostly due to only one of the several subjects' values being above the MDL. For all of the control rats, none of the mean values of the sum of the amount of Ce in the organs or blood exceeded 1% of the nano-CeO2 dose given to the paired nano-CeO2 treated rats.
Nine values from nano-CeO2 treated subjects were outliers by the Grubb's test. Of the 462 samples from nano-CeO2 treated rats analysed for Ce, all but 56 (14%) were above the MDL; 20 were brain, 16 blood, and 15 were skeletal muscle samples, indicating the lowest nano-CeO2 distribution into those organs.
According to the authors, there were no statistically significant differences in the percentage of the infused nano-CeO2 dose between those rats that received a single dose of Ce5 and those that received 5 daily consecutive infusions of the same Ce5 dose. However, based on the figure available, the percentage of Ce5 dose in blood was higher in rats treated once with nano-CeO2 than in rats exposed 5 times to Ce5 (see in Table 2 below). The order of tissue concentration for Ce5 was as follows 30 days after single or repeated infusion: liver > bone marrow > spleen ≥ skeletal system ≥ lung > skeletal muscle ≥ fat ≥ kidney > heart > thymus > blood > brain.
Yokel RA et al. described that the infusion of 6mg Ce30/kg significantly increased cerium in most organs and blood and that this was shown by the statistically significant differences compared to control rats (data on controls not shown) and when all of the cerium levels in the control rats were below the MDL, leading to the inability to statistically compare results from control and nano-CeO2 treated rats. However, based on the corresponding figure, the cerium content (expressed as % of nano-CeO2 dose) was significantly increased only in liver at 1 h and 90 days post-infusion and in spleen at 90 days post-infusion (see in Table 2 below). The only statistically significant decreases of cerium seen up to 90 days after infusion of Ce30 (6 mg/kg) were in the liver and skeletal muscle. One hour after a single i.v. infusion of Ce30 at 6 mg/kg, 71% of the dose was in the liver. The percentage of the injected dose in the liver 90 days after the single i.v. infusion had decreased to 26%. The order of tissue concentration for Ce30 was as follows:
* 1 h after infusion: liver > spleen > lung > bone marrow > skeletal system ≥ skeletal muscle > blood > lymph node ≥ kidney ≥ heart = thymus > brain > adrenal;
* 30 d after infusion: liver > bone marrow ≥ spleen > skeletal system > lung ≥ skeletal muscle ≥ kidney ≥ heart ≥ blood ≥ lymph node ≥ brain > adrenal ≥ thymus;
* 90 d after infusion: liver > bone marrow ≥ spleen ≥ skeletal system > skeletal muscle ≥ lung ≥ lymph node ≥ kidney ≥ blood = thymus ≥ brain ≥ heart ≥ adrenal.
CeR distribution was generally similar to those of Ce5 and Ce30, as well as between CeR doses of 20 and 50 mg/kg (see in Table 3 below):
* 1 h after infusion of 50 mg/kg: liver > bone marrow = lung ≥ spleen > skeletal muscle > skeletal system ≥ blood ≥ thymus > brain;
* 30 d after infusion of 50 mg/kg: bone marrow > liver ≥ spleen > skeletal system > lung = skeletal muscle > lymph node > brain = blood = thymus;
* 30 d after infusion of 20 mg/kg: liver > spleen ≥ bone marrow > skeletal system > skeletal muscle ≥ lung > fat > blood > thymus ≥ brain.
There was no significant reduction of cerium levels at 30 days, compared to the first hour, after infusion of CeR at 50 mg/kg. In contrast, cerium increased in bone marrow and spleen between 1 hour and 30 days post-infusion, resulting in a greater percentage of the dose in these mononuclear phagocyte system (MPS) sites than the two other nano-CeO2 treatments.
Results of the relative deposition index showed accumulation in liver within 1 h that persisted for 30 or 90 days for Ce5 and Ce30, but not for CeR (Table 4). According to the authors, the accumulation of Ce5 and CeR in the bone marrow at 30 days and CeR in the spleen at 30 days might represent uptake of nano-CeO2 that was located in other sites, or dissolution of nano-CeO2 and distribution of cerium to these sites.
Yokel RA et al. added that the sum of the percentages of the CeO2 dose in the sampled blood and organs accounted for 70 and 72% of single
and repeated Ce5; 79, 56 and 36% of Ce30 at 1, 30 and 90 days; 53 and 78% for the 50 mg/kg CeR dose after 1 h and 30 days; and 49% for the 20 mg/kg CeR dose after 30 days. Since previous publications described an absence of faecal and urinary cerium excretion (Hirst SM et al., 2011; Yokel RA et al., 2012), the authors suggested that the remainder of the dose could be in un-sampled sites, one of which appeared to be adherence to the luminal wall of blood vessels as demonstrated by Dan M et al. (2012). - Details on excretion:
- No data available
- Metabolites identified:
- not measured
- Bioaccessibility (or Bioavailability) testing results:
- No data available
- Conclusions:
- Cerium determined in about 12 organs and tissues from 1 h to 90 days after i.v. infusion of a 5-nm polyhedral, a 30-nm cubic nano-CeO2, and a CeO2 nanorod was mainly in mononuclear phagocyte system (MPS) organs, with no evidence of entry into brain parenchyma. There was little decrease in the percentage of nano-CeO2 dose in the rat for up to 90 days, although there was a decrease in the liver and increase in some other sites, suggesting some translocation or nano-CeO2 dissolution and cerium redistribution.
These results suggested that size, shape, doses, and dosing schedules did not have a profound effect on the biodistribution or persistence of tested nano-CeO2, and the distribution and fate of these representative nano-CeO2 and others previously studied by Yokel RA et al. were more similar than different.
=> Interpretation of results: bioaccumulation potential in reticulo-endothelial organs (liver and spleen) - Executive summary:
Yokel RA et al. (2014) aimed at determining if the biodistribution, persistence, and toxicity of nano-CeO2 were affected by dosing schedule, dose, or particle shape.
Citrate-coated nano-CeO2 with various physico-chemical properties were selected and characterised:
Parameters
Results
Methods
Ce5
Ce30
CeR
Primary particle size
Expected value
Measured value
5 nm
4.6 ± 0.1 nm
30 nm
31.2 ± 0.5 nm
-
Average diameter of 9.9 ± 2.0 nm and length of 264 ± 112 nm
-
TEM
Particle size distribution (volume-average)
7 nm in water
41 nm in water
Bimodal: 100 nm for 5% and 400 nm for 95% in 10% sucrose(a)
Dynamic light scattering (DLS)
Stability
Slight agglomeration/aggregation tendency
Slight agglomeration/aggregation tendency
Slight agglomeration/aggregation tendency
-
Specific surface area
121 m²/g
15 m²/g
54 m²/g (estimated from diameter, length & density)
BET method
Surface charge in water at pH ~7.3 (zeta potential)
-53 ± 7 mV
-56 ± 8 mV
-25 mV(b)
DLS
Shape
Polyhedral
Cubic
Rod
TEM
Crystallinity
Crystalline
Crystalline
Crystalline
XRD, TEM
Cerium content
in nano-CeO2 suspension
Analytical dose was 85% of the intended 100 mg/kg nominal dose.
85% of the intended 100 mg/kg nominal dose.
No data
ICP-MS
Surface properties –
Extent of surface
citrate coating (as % of monolayer)
ca. 40%
ca. 18%
ca. 100%
No data
Free Ce content
in suspensions
11.6 ± 0.3%
<< 1%
No data
ICP-MS
pH of suspensions
Maintained at pH 7.7 to 8
pH 3.9
No data
pH metry
(a): Measured on the original sample that was stored three years in 10% sucrose suspension
(b): An experimental study of zeta potential measurements on short nanorods (L/D ~ 4) showed that their expected hydrodynamic diameter was 10% higher than that of spherical particles. This correction was applied to the obtained DLS results.
Ce5 and Ce30 were infused in water to avoid agglomeration. A concurrent infusion was given of 1.8% sodium chloride at the same rate in a second i.v. cannula to deliver a net isosmotic load to the rat. The dried CeR sample was suspended in 10% sucrose solution by sonication for 10 min and was infused into the rats. All nano-CeO2 dispersions were infused at a rate of 2 mL/kg rat body weight over 1 h, with the exception of the 50 mg/kg dose of nanorods, which was infused over 2.5 h.
Ce5 at 11 mg/kg, Ce30 at 6 mg/kg, and CeR at 20 and 50 mg/kg were i.v. infused into male rats once (3 to 9/gp).The Ce5 dose of 11 mg/kg was also infused daily for 5 consecutive days to male rats (5/gp). Control rats were infused with the vehicle. Rats exposed once or repeatedly to Ce5 were sacrificed 30 days after i.v. infusion. Ce30-exposed rats were sacrificed 1 h, 30 and 90 days after i.v. infusion, while rats treated once with CeR were terminated 1 h and 30 days after i.v. infusion. Cerium was determined in multiple organs and blood using inductively coupled plasma mass spectrometry (ICP-MS). The method detection limits (MDL) were 0.089 mg Ce/kg for tissues and 0.018 mg Ce/L in blood samples. Spike recovery for ICP-MS analyses was 94 ± 3%. Average relative percent difference between replicate analyses was 3.2 ± 2.0%. Ce concentrations below the MDL were assigned 50% of the MDL and included in the statistical analysis as such.
Compared to vehicle-infused controls, elevated cerium was seen in numerous tissues from CeO2-treated rats, with no evidence of entry into brain parenchyma, and that regardless of size, dose, dosing schedule, and shape of the infused nano-CeO2. According to the authors, the cerium in the brain of the CeO2-treated rats could be accounted for by the cerium in circulating blood. Liver, spleen, and bone marrow (mononuclear phagocyte system (MPS) components), contained the largest percentage of the dose. When normalized to dose, and compared to results of prior work with these nano-CeO2, the distribution and retention of repeated and lower doses of Ce5, Ce30 and CeR were not greatly different from much higher doses of Ce5 and Ce30. Higher doses resulted in a greater percentage of uptake by the spleen and bone marrow and a greater percentage of CeR dose in the bone marrow 30 days after its administration than the other nano-CeO2.
The authors noted that 85% of the samples, including those collected 90 days after the single nano-CeO2 administration, were above the MDL, while only 20% of the samples from non-CeO2 treated rats were above the Method Detection Limit (MDL) of this highly sensitive analytical method (e.g., liver, spleen, brain, bone marrow, bone, and lung).
Overall these results suggested the biodistribution and retention of cerium after i.v. administration of different sizes, doses, dosing schedules, and nano-CeO2 shapes were more similar than different.
- Endpoint:
- basic toxicokinetics in vivo
- Type of information:
- experimental study
- Adequacy of study:
- weight of evidence
- Study period:
- Not applicable
- Reliability:
- 2 (reliable with restrictions)
- Rationale for reliability incl. deficiencies:
- study well documented, meets generally accepted scientific principles, acceptable for assessment
- Remarks:
- but test not conducted under GLP conditions.
- Reason / purpose for cross-reference:
- reference to other study
- Objective of study:
- distribution
- other: nano-CeO2 concentration, localization and chemical speciation in the brain
- Qualifier:
- no guideline followed
- Principles of method if other than guideline:
- - Unique intravenous (i.v.) injection of a suspension of nanometric cerium dioxide (nano-CeO2) in rats; sacrifice of animals 1 and 20 hours after injection in the study of 2010 and 30 days after injection in the study of 2012
- Distribution in whole blood, brain cortex, spleen, and liver: inductively coupled plasma mass spectrometry (ICP-MS)
- Localization in hippocampus, cerebellar hemisphere, heart, spleen, liver, lung, and kidney: light and electron microscopy (LM and EM)
- Nano-CeO2 concentration, localization, and chemical speciation in the brain (e.g., hippocampus, cerebellar hemisphere):
* ICP-MS
* LM and EM
* electron energy loss spectroscopy (EELS)
- Blood-brain barrier (BBB) integrity: brain perfusion of BBB permeability or vascular space markers and quantification of signal using fluorescence or ICP-MS - GLP compliance:
- not specified
- Remarks:
- The GLP status was not specified in the article.
- Specific details on test material used for the study:
- - Name of test material: Nanometric cerium dioxide (citrate-coated nano-CeO2)
- Supplier: None (in-house synthesis using a hydrothermal method from cerium chloride heptahydrate)
- Substance type: Monoconstituent substance
- Substance form: Nanoparticulate substance / crystalline nanomaterial in suspension
- Primary particle size (HRTEM/HRSTEM): ca. 5 nm
- Particle size distribution (DLS): Bimodal with two peaks at 6.4 and 12.6 nm in water (pH 8 and 37°C) and > 70% of non-agglomerated particles
- Stability: No agglomeration/aggregation in water at pH 7.7-8 (due to citrate stabilisation) based on zeta potential value, TEM observations and particle size distribution; the portion of the dose of non-agglomerated 5-nm particles was 98 vol% for the as-synthesised particles (87 vol% in 0.9% NaCl solution). At pH below 7, nano-CeO2 agglomerated.
- Specific surface area (BET): 121 m²/g (assuming a density of 7600 kg/m3, the corresponding average diameter should be 6.5 nm)
- Surface charge (electrophoretic mobility measurements): Zeta potential of -53 ± 7 mV in water (pH ~7.35)
- Isoelectric point: No data available
- Shape (HRTEM/HRSTEM, EDX): Polyhedral
- Crystallinity (HRTEM/HRSTEM, EDX, XRD): Face-centred cubic
- Analytical purity / impurities (ICP-MS): Traces of lanthanum (< 10E-5 wt%)
- Number density of nano-CeO2 in the suspension: 8.7 x 10E16 particles/mL (assuming monodispersde spheroids with a diameter of 5 nm and a specific gravity of 7.65 for crystalline CeO2)
- Cerium content in nano-CeO2 suspension (ICP-MS): 4.35 ± 0.20%
- Solubility: Described as essentially insoluble in water
- Oxidation degree (EELS): High Ce(III) valence
- Surface properties (TGA): Citrate coating for stabilisation of nano-CeO2 in suspension
- Lot/batch No.: No data available
- Expiration date of the lot/batch: No data available
- Other information: The number-based mean CeO2 hydrodynamic diameter in suspension was of ca. 7nm. CeO2 suspension contained 11.6% free cerium ions.
Further explanations on the physico-chemical characterisation of CeO2 nanoparticles are presented below in "any other information on materials and methods incl. tables". - Radiolabelling:
- no
- Species:
- rat
- Strain:
- Sprague-Dawley
- Sex:
- male
- Details on test animals or test system and environmental conditions:
- TEST ANIMALS
- Source: University of Kentucky (USA) in the study of 2010; Harlan (USA) in the study of 2012
- Age at study initiation: No data available
- Weight at study initiation: From 324 ± 29 g (in 2010) to 328 ± 21 g (in 2012)
- Fasting period before study: No data available
- Housing: Individually
- Individual metabolism cages: No data available
- Diet: Ad libitum, 2018 Harlan diet according to Hardas SS et al. (2012)
- Water: Ad libitum, RO water according to Hardas SS et al. (2012)
- Acclimation period: No data available
ENVIRONMENTAL CONDITIONS
- Temperature: ca. 21.1°C according to Hardas SS et al. (2012)
- Humidity: 30-70% according to Hardas SS et al. (2012)
- Air changes: No data available
- Photoperiod : 12 hrs dark / 12 hrs light according to Hardas SS et al. (2012)
IN-LIFE DATES: No data available - Route of administration:
- intravenous
- Vehicle:
- water
- Remarks:
- adjusted to pH 8
- Details on exposure:
- Rats were prepared with two cannulas, surgically inserted into femoral veins, which terminated in the vena cava. The next day non-anaesthetized rats were infused i.v. via the shorter cannula with nano-CeO2 as a ~4% suspension in water or water (controls) adjusted to pH 8. To compensate for the i.v. administration of a considerable volume of water or grossly hypotonic nano-CeO2 suspension, concurrent i.v. infusion of an equal volume and rate of 1.8% sodium chloride in water was delivered into the second, longer, cannula. Based on the intended concentration of the CeO2 in suspension of 5%, it was infused at around 0.6 mL/h. The 100, 175, and 250 mg/kg CeO2 doses were delivered over 1, 1.75, and 2.5 h, in total volumes of ~0.6, 1.05, and 1.5 mL, respectively.
In the study from 2012, the authors detailed that the suspended nano-CeO2 were i.v. infused over 1 h. - Duration and frequency of treatment / exposure:
- A single exposure (sacrifice 1 and 20 hours after infusion or 30 days after infusion)
- Dose / conc.:
- 0 mg/kg bw (total dose)
- Remarks:
- (control) in both initial and main studies
- Dose / conc.:
- 100 mg/kg bw (total dose)
- Remarks:
- in both initial and main studies
basis: nominal nano-CeO2 dose
This corresponded to an analytical dose of 85 mg/kg. - Dose / conc.:
- 175 mg/kg bw (total dose)
- Remarks:
- in the initial study only
basis: nominal nano-CeO2 dose - Dose / conc.:
- 250 mg/kg bw (total dose)
- Remarks:
- in the initial study only
basis: nominal nano-CeO2 dose - No. of animals per sex per dose / concentration:
- - Initial study
* rats + 0 mg/kg (water - controls) => n ≥ 6
* rats + 100 mg/kg => n ≥ 1
* rats + 175 mg/kg => n = 3
* rats + 250 mg/kg => n = 5
- Main study
(a) Rats terminated at 1 h (2010):
* rats + 0 mg/kg (water - controls) => n = 7
* rats + 100 mg/kg (nominal) => n = 11
(b) Rats terminated at 20 h (2010):
* rats + 0 mg/kg (water) => n = 8
* rats + 100 mg/kg (nominal) => n = 12
(c) Rats terminated at 30 d (2012):
* rats + 0 mg/kg (water) => n = 7
* rats + 85 mg/kg (analytical) => n = 9 - Control animals:
- yes, concurrent vehicle
- Details on study design:
- In the study from 2010, CeO2 content of the suspension, which was prepared with an intended concentration of 5% CeO2, was determined by digestion of nano-CeO2 suspension samples and analysis by ICP-MS. Analysis of nano-CeO2 suspension (digested in HNO3/H2O2) by ICP-MS showed that the CeO2 content of the as-synthesised suspension was 4.35 ± 0.20%. Therefore, the actual dose was ca. 85 mg/kg, rather than the intended 100 mg/kg.
Hardas SS et al. explained that rats were terminated 1 and 20 h after completion of the infusion to enable determination of acute and later effects of nano-CeO2 on oxidative stress parameters and potentially initial translocation and/or clearance of the nanoparticles. - Details on dosing and sampling:
- PHARMACOKINETIC STUDY (Absorption, distribution, excretion)
- ORGAN WEIGHT AND DISTRIBUTION:
Brain, heart, spleen, liver, lung, and right kidney were weighed. Samples of whole blood, brain (cortex, hippocampus, cerebellum), spleen (medial tip), and liver (near tip of larger of the two bifurcated lobes) were collected 1 and 20 h after the single i.v. injection for cerium (Ce) determination by ICP-MS (after microwave digestion), and for LM and EM analyses. The detection limit of this method (MDL) was 0.089 mg Ce/kg. Ce concentration below the MDL was reported as 50 % of the MDL.
- NANO-CeO2 ACCUMULATION IN THE BRAIN:
Samples of hippocampus and cerebellar hemisphere were collected in neutral buffered formalin for LM and EM for oxidative stress assessment.
Coronal brain slices containing hippocampi were cut and immediately immersed in 4% buffered formalin fixative overnight and stored in PBS at 4°C. The isolated hippocampi were sliced further into 3 mm3 pieces, dehydrated, and embedded in Araldite 502. One-micrometre-thick sections were cut and stained with toluidine blue for light microscopic screening and examination. Thin sections from selected blocks were collected on copper grids for TEM evaluation. Additional sections collected on carbon-coated grids were prepared for high-resolution TEM (HRTEM), STEM and EDX analyses.
Determination of CeO2 oxidation degree by EELS was conducted in the brain of rats after its i.v. infusion. The M5 and M4 CeO2 edges at 883 and 901 Ev and their heights and edge splitting were determined as measures of Ce(III) and Ce(IV), respectively.
OTHER
- BRAIN-BLOOD BARRIER (BBB) INTEGRITY:
Rats were given i.v. in saline, 5 min before termination, Na fluorescein (334 Da) and horseradish peroxidase (HRP; ca. 44,400 Da) as small and large BBB permeability markers, respectively. Twenty-five minutes prior to the BBB marker infusion, rats were given 5 mg/kg diphenhydramine intraperitoneally (ip) to block the histamine response to HRP. Post-mortem brain cortex was obtained to quantify fluorescein and HRP. - Statistics:
- The slot blot, Western blot, and enzyme assay results were presented as mean ± SEM. The control mean was normalised to 100%.
Grubb's test was used to identify outliners in the BBB permeability marker results. Student's unpaired t-test was used to evaluate significant difference between controls and CeO2 treated samples. Significance was accepted at p < 0.05. - Preliminary studies:
- Hardas SS et al. conducted an initial study in which rats were exposed to nano-CeO2 (0, 100, 175 or 250 mg/kg).
Among rats exposed to 175 and 250 mg CeO2/kg, three animals died. Two of the five rats that received 250 mg CeO2/kg died 15 and 50 min after the CeO2 infusion, demonstrating significant respiratory distress, fluid exudation from the nose, and seizures. One of the three rats that received 175 mg CeO2/kg died 1 h after the infusion, demonstrating fluid in its stomach and pleural cavity. All demonstrated lethargy and/or mild respiratory distress. Post-mortem examination of the distressed rats often showed pulmonary haemorrhagic foci and extravasation into the surrounding parenchyma. One rat that received 100 mg CeO2/kg and six controls showed no distress.
Therefore, the nominal dose tested in the main study was 100 mg CeO2/kg. - Details on absorption:
- Hardas SS et al. demonstrated that nano-CeO2 was rapidly cleared from blood following a single i.v. exposure (i.e., 1 or 20 h later).
- Details on distribution in tissues:
- - ORGAN WEIGHT AND DISTRIBUTION:
One or twenty hours after injection, there were no significant differences in organ weights between the CeO2-infused and control groups except for the spleen, which weighed significantly more (0.73 ± 0.11 vs. 0.56 ± 0.16 g) in the treated vs. control rats terminated at 20 h. In contrast to the lack of significant CeO2 accumulation in the brain, considerable nano-CeO2 was seen in the liver and spleen, as demonstrated by Ce concentration analyses by ICP-MS and EM (see in Table 1 below). Nano-CeO2 was detected in Kupffer cells along the sinusoidal surface and in some hepatocytes. Only rare nano-CeO2 was localized to the brain vascular compartment, but no CeO2 was observed in cerebellum or hippocampus by electron microscopy.
Thirty days after injection, observations were quite similar as that described above: 30 days after infusion into rats, the 5-nm CeO2 agglomerates were located in the liver tissue; in contrast, a very small amount of CeO2 was present in the brain or in the blood compared to the liver (see in Table 2 below).
- NANO-CeO2 ACCUMULATION IN THE BRAIN:
One or twenty hours after injection, no nano-CeO2 was found in the hippocampus (astrocytes, adjacent pericytes, or surrounding neurites). Other areas examined included the cerebellum and paraventricular organs such as medium eminence. Again, no nano-CeO2 was found. Based on Ce concentration estimation and extensive EM imaging, the brain CeO2 accumulation appeared to be largely limited to the vascular compartment.
An evaluation of nano-CeO2 oxidation degree in the brain tissue revealed that it was similar to the as-synthesised particles (i.e., high Ce(III) valence), indicating that nano-CeO2 was not significantly changed after its biodistribution.
Again, 30 days after injection, observations were quite similar as that described above: the particles rather located on the luminal side of the BBB endothelial cells. The high Ce(III)/Ce(IV) ratio, obtained in the as-synthesised particles, seemed to have only been slightly altered in liver after 30 days. However, this difference was not significant (data not shown).
- BBB INTEGRITY:
Regardless of the sacrifice time point, no evidence of BBB penetration of nano-CeO2 was seen and no sign of alterations in BBB integrity was found. - Details on excretion:
- No data available
- Metabolites identified:
- not measured
- Bioaccessibility (or Bioavailability) testing results:
- No data available
- Conclusions:
- Regardless of the sacrifice time point, i.v. administered nano-CeO2 did not appear to cross or alter the BBB and thus did not accumulate in the brain. These i.v. infused nanoparticles remained in the mammal model, rather in reticulo-endothelial organs such as spleen or liver.
=> Interpretation of results: no bioaccumulation potential into brain but bioaccumulation into liver and spleen - Executive summary:
Hardas SS et al. (2010, 2012) aimed at characterising the biodistribution of a suspension of 5-nanometer cerium dioxide (nano-CeO2) from blood into brain up to 30 days after systemic administration.
A water or saline suspension of 4.35% crystalline nano-CeO2 (8.7 x 10E16 particles/mL) was used in this study. The in-house synthesised nano-CeO2 had a primary particle size of ~5 nm, a polyhedral shape, a crystalline structure, a specific surface area of 121 m²/g and a high Ce(III) valence. The particles showed no or few agglomeration/aggregation tendency in aqueous solutions at physiological pH, probably due to citrate coating which stabilised the suspended particles. The zeta potential of the nano-CeO2 was of -53 mV, at pH 7.35. Except traces of lanthanum, no contamination was detected in the nano-CeO2 suspension.
In a first study (2010), the nano-CeO2 suspension was intravenously (i.v.) infused into Sprague-Dawley male rats (0, 100, 175, or 250 mg/kg as nominal dose), which were terminated after 1 or 20 h. In the second study (2012), the nano-CeO2 suspension was i.v. infused (0 or 85 mg/kg, as measured dose) into male Sprague-Dawley rats which were terminated 30 days later. CeO2 concentration, localization, and chemical speciation in the brain, blood, liver and spleen were assessed by inductively coupled plasma mass spectrometry (ICP-MS), light (LM) and electron microscopy (EM), and electron energy loss spectroscopy (EELS). Na fluorescein and horseradish peroxidase (HRP) were given i.v. as blood-brain barrier (BBB) integrity markers.
In the first study, mortality was seen after administration of 175–250 mg CeO2/kg. However, given i.v. at 100 mg/kg (nominal dose), no systemic effect and no significant differences in organ weight were found between CeO2-infused and control rats, except for the spleen. CeO2 accumulation was seen in the liver and spleen, but not in the brain. Indeed, twenty hours after infusion of 100 mg CeO2/kg, brain HRP was globally unchanged, showing a probable lack of BBB penetration. EM and EELS revealed a high Ce(III) valence in nano-CeO2 agglomerates in the brain vascular compartment. Nano-CeO2 was not seen in microvascular endothelial or brain cells. These results suggested that in the brain, most of the nano-CeO2 was located on the luminal side of the BBB endothelial cells.
In the second study (2012), the BBB was visibly intact and no nano-CeO2 was seen in the brain cells at day 30 post-infusion. The particles located on the luminal side of the BBB endothelial cells. Moreover, as observed in the previous study, nano-CeO2 was found accumulated in peripheral organs like the liver. EELS analysis showed that even after a long-term exposure time of 30 days nano-CeO2 continued to display a significant +3 valence on the surface.
In conclusion, the CeO2 nanoparticles remained in the mammal model, mainly in reticulo-endothelial organs, up to 30 days after its i.v. administration but did not cross the BBB.
- Endpoint:
- basic toxicokinetics in vivo
- Type of information:
- experimental study
- Adequacy of study:
- weight of evidence
- Study period:
- not applicable
- Reliability:
- 2 (reliable with restrictions)
- Rationale for reliability incl. deficiencies:
- study well documented, meets generally accepted scientific principles, acceptable for assessment
- Remarks:
- ; however, the physico-chemical characterisation was performed on non-radioactive nano-CeO2 instead of the radioactive nanoparticles which were the material administered to animals.
- Reason / purpose for cross-reference:
- reference to same study
- Reason / purpose for cross-reference:
- reference to same study
- Reason / purpose for cross-reference:
- reference to other study
- Objective of study:
- bioaccessibility (or bioavailability)
- distribution
- excretion
- other: clearance
- Qualifier:
- no guideline followed
- Principles of method if other than guideline:
- - Administration of a suspension of nanometric cerium dioxide (nano-CeO2) by intratracheal (IT) instillation to rats; sacrifice of animals 5 min, 2, 7 and 28 days post-dosing
- Bioaccessibility: Scanning electron microscopy (SEM), analytical ultracentrifugation (AUC), laser diffraction (LD), inductively coupled plasma-mass spectrometry (ICP-MS)
- Biodistribution, clearance, excretion: Radioactivity measurement using a gamma counter - GLP compliance:
- not specified
- Remarks:
- The GLP status was not specified in the article.
- Radiolabelling:
- yes
- Remarks:
- neutron-activated 141CeO2
- Species:
- rat
- Strain:
- Wistar
- Sex:
- male
- Details on test animals or test system and environmental conditions:
- TEST ANIMALS (both studies)
- Source: Charles River Laboratories (USA)
- Age at study initiation: 8 weeks old
- Weight at study initiation, fasting period before study: No data available
- Housing: In standard microisolator cages
- Individual metabolism cages: No data available
- Diet: Ad libitum; commercial chow (PicoLab Rodent Diet 5053, USA)
- Water: Ad libitum; reverse-osmosis purified water
- Acclimation period: At least 7 days before the start of experiments
ENVIRONMENTAL CONDITIONS
- Temperature, humidity: Controlled conditions of temperature and humidity
- Air changes: No data available
- Photoperiod: Controlled conditions of light
IN-LIFE DATES: No data available - Route of administration:
- intratracheal
- Vehicle:
- other: sterile distilled water
- Details on exposure:
- PREPARATION OF DOSING SOLUTIONS (both studies):
Particle suspensions at specified concentrations were prepared in sterile distilled water in conical polyethylene tubes. A critical sonication energy to
achieve the smallest particle agglomerate size was used. The suspensions were sonicated at in a cup sonicator. The sample tubes were immersed in running cold water to minimize heating of the particles during sonication. The hydrodynamic diameter (HD), polydispersity index (PdI) and zeta potential (ζ) of each suspension were measured by dynamic light scattering (DLS). In addition, the radioactivity in multiple aliquots of each suspension or solution was measured in a Gamma Counter.
VEHICLE
- Concentration in vehicle: 0.67 mg/mL
- Lot/batch no., purity: No data available
HOMOGENEITY AND STABILITY OF TEST MATERIAL: No data available
INTRATRACHEAL ADMINISTRATION
Each rat was anaesthetized with isoflurane. The 141nano-CeO2 suspension was delivered to the lungs through the trachea. Each rat was then placed in a metabolic cage with food and water ad libitum for faecal and urine sample collection. Rats (5/gp) were humanely killed 5 min, 2, 7 and 28 days post-dosing. - Duration and frequency of treatment / exposure:
- A single exposure (sacrifice 5 min, 2, 7 and 28 days post-dosing in both studies)
- Dose / conc.:
- 0 mg/kg bw (total dose)
- Remarks:
- (control)
- Dose / conc.:
- 1 mg/kg bw (total dose)
- Remarks:
- basis: nominal nano-CeO2 conc (both studies).
- No. of animals per sex per dose / concentration:
- At least 5
- Control animals:
- yes, concurrent vehicle
- Details on study design:
- - Dose selection rationale: The authors first evaluated pulmonary responses to i.t.-instilled nano-CeO2 and observed dose-dependent inflammatory effects. This experiment was performed to determine the safe dose for i.t. instillation of nano-CeO2 where inflammation or injury was minimal. Twenty-four rats (wt. = 249 ± 4 g) were i.t. instilled with CeO2 suspension at 0.2 or 1.0 mg/kg (n = 6/gp). Another group of rats instilled with an equivalent volume of distilled water served as controls. The particle suspensions were delivered to the lungs through the trachea. Twenty-four hours later, rats were anaesthetized and then euthanized via exsanguination, with a cut in the abdominal aorta. The trachea was exposed and cannulated. The lungs were then lavaged 12 times with PBS. The cells from all washes were separated from the supernatant by centrifugation. Total cell count and haemoglobin measurements were made from the cell pellets. A dilute cell suspension was cytocentrifuged, the cytospin was stained and differential cell counting was performed. The supernatant from the first two washes was clarified via centrifugation, and used for standard spectrophotometric assays for lactate dehydrogenase (LDH), myeloperoxidase (MPO) and albumin.
- Rationale for animal assignment: No data available - Details on dosing and sampling:
- PHARMACOKINETIC STUDY (Absorption, distribution, excretion) (both studies)
Analysis of rats at 5 minutes post-i.t. instillation was performed to obtain an accurate measure of the initial deposited dose.
- Tissues and body fluids sampled: urine, faeces, blood, plasma, serum, lung, bone marrow, femoral bone, skin, brain, skeletal muscle, testicles, kidneys, spleen, heart, liver, stomach, small intestine, large intestine, caecum, gastro-intestinal tract (GIT)
- Time and frequency of sampling: Twenty four-hour samples of faeces and urine were collected at selected time points (0–24 hours, 2–3 days, 6–7 days, 9–10 days, 13–14 days, 20–21 days and 27–28 days post-i.t. instillation). The other tissues were sampled 7 and 28 days post-administration.
At each time point, rats were anaesthetized and as much blood as possible was collected from the abdominal aorta. Plasma and red blood cells were separated by centrifugation. Each sample weight was recorded. Radioactivity was measured in a Gamma Counter. Disintegrations per minute were calculated from the measured counts per minute (minus background) and the counter efficiency. Data were expressed as μCi/g and as a percentage of the administered dose retained in each organ. All radioactivity data were adjusted for physical decay over the entire observation period. The radioactivity in organs and tissues not measured in their entirety was estimated from measured aliquots as a percentage of total body weight as follows: skeletal muscle, 40%; bone marrow, 3.2%; peripheral blood, 7%; skin, 19%; and bone, 6%.
- Other:
> To determine the pulmonary distribution of instilled 141nano-CeO2 within the lungs at 1 d post-instillation, a separate cohort of rats were i.t.-instilled with 1 mg/kg 141nano-CeO2. Twenty-four hours later, the lungs were lavaged. The BAL fluid was centrifuged to separate lavaged cells from the supernatant. The cell pellets were resuspended in PBS. The lavaged lungs, BAL supernatants and cell pellets were analysed for 141Ce. The total radioactivity in each of the three lung compartments was expressed as a percentage of the total radioactivity recovered in the whole lungs (Molina et al., 2014).
> Assessment of alveolar macrophage uptake of nanoceria in vivo (Konduru et al., 2015): Non-radioactive CeO2 NPs were instilled in a separate cohort of rats at the same dose and concentration (1 mg/kg). At 1 or 5 days post-instillation, rats were sacrificed and their lungs lavaged. BAL cells were cytocentrifuged and fixed on microscope slides. Uptake of nanoceria by cells was analyzed by microscopy. Each macrophage was scored for the presence of internalized NPs.
> Assessment of pulmonary effects of CeO2 nanoparticles – Bronchoalveolar lavage and analyses (Konduru et al., 2015):
This experiment was performed to to identify a safe dose for pharmacokinetic studies on instilled materials. Thirty five rats were instilled intratracheally with CeO2 NP suspensions at 0 (control, distilled water), 0.2, 1.0, and 5 mg/kg (n = 5 rats/group). Twenty-four hours later, rats were euthanized via exsanguination, and the trachea was exposed and cannulated. The lungs were then lavaged 12 times with 3 mL of Ca++- and Mg++-free 0.9 % sterile PBS. The cells from all washes were separated from the supernatant by centrifugation (350 x g at 4 °C for. Total cell count and hemoglobin measurements were made from the cell pellets. A dilute cell suspension was cytocentrifuged, the cytospin was stained, and differential cell counting was performed. The supernatant from the first two washes was clarified via centrifugation and used for standard spectrophotometric assays for LDH, MPO, and albumin [65].
OTHER
IN VITRO BIOACCESSIBILITY TESTS (Molina et al., 2014):
Nano-CeO2 was incubated under varying pH and chemical conditions to measure bioaccessibility, which is the fraction of cerium (Ce) that dissolves. The nanoparticles were incubated in different fluids at 37 °C: phosphate-buffered saline (PBS) for 28 days to simulate surface deposition in the lungs, synthetic phagolysosomal simulant fluid (PSF) for 28 days to simulate uptake and digestion in macrophages, 0.1N HCl for 1 day to simulate oral ingestion and stomach transit time, and fasted state simulant intestinal fluid (FaSSIF) for 7 days to simulate gastro-intestinal (GI) intraluminal conditions. All incubation times were chosen at or above the maximum realistic residence time of nano-CeO2 in specific body compartments, since the authors expected low dissolution in these simulant fluids. Nanoparticle agglomeration (size and charge), release of ions in supernatant by inductively coupled mass spectroscopy (ICP-MS), structural changes of particles by scanning and transmission electron microscopy (SEM/TEM) on sediment and nanoparticle durability by selected area electron diffraction (SAD) were all determined. - Statistics:
- All data were analysed using multivariate analysis of variance (MANOVA) followed by Bonferroni (Dunn) post hoc tests.
- Preliminary studies:
- Prior to pharmacokinetic experiments, Molina RM et al. determined a dose for i.t. instillation that would not cause significant pulmonary injury and inflammation. The authors focused on evaluation of acute effects measured at 24 h post-instillation (n = 6/gp). No significant increase was found in several endpoints measured in bronchoalveolar lavage (BAL) at 0.2 and 1 mg/kg of nano-CeO2 compared with vehicle control. The only parameter that significantly decreased was the macrophage number: ~9 x 10E6 macrophages in BAL of controls vs. ~6 x 10E6 and ~5 x 10E6 macrophages in BAL of rats treated with 0.2 and 1 mg/kg nano-CeO2, respectively. The significant decrease in macrophages retrieved in BAL from nano-CeO2-instilled animals could have been due to macrophage toxicity or enhanced macrophage adhesion on the epithelial surface and thus reduced recovery by lavage. No significant changes in lavaged neutrophil, lymphocyte, or eosinophil numbers, and LDH, MPO, albumin and haemoglobin levels were observed following instillation of nano-CeO2. Thus, i.t.-instilled nano-CeO2 did not induce lung injury and inflammation up to 1mg/kg.
However, pulmonary responses to instilled CeCl3 were observed at the lower 0.1 mg/kg dose. There were significant increases in neutrophils, MPO and LDH and a significant decrease in albumin.
In the Konduru et al. (2015) study, CeO2 NPs induced a dose-dependent injury and inflammation as indicated by increased neutrophils in the BAL fluid at 24 h post-instillation, that was statistically significant only at 5.0 mg/kg, and decreased at 5 days post exposure. At this dose only, CeO2 NP also increased the levels of myeloperoxidase (MPO) and albumin (according to the authors; data not shown for both paramters) and lactate dehydrogenase (LDH). No change in the LDH level was measured at day 5. The numbers of lavaged macrophages increased slighlty (non significant) at the highest dose of CeO2 NP 1 day after the exposure and showed a slight increase at day 5. - Details on absorption:
- The translocated Ce from nano-CeO2 at 28 days was very low (0.86% of dose).
Nano-CeO2 bioavailability from the lungs was orders of magnitude higher than that via the gut (see data in "Nano-CeO2 - biodistribution in rats - oral - V2 2014Moli"). - Details on distribution in tissues:
- Molina et al., 2014: The authors examined the distribution of 141Ce within the lungs at 24 hours post-instillation of nano-CeO2 or CeCl3. The majority of nano-CeO2 (68.6%) was detected in the lavaged lungs representing adhered and tissue-associated 141Ce. Approximately 26% of nano-CeO2 was measured in the cell pellet, presumably mostly in macrophages. Only 5.7% of recovered 141Ce was found in the supernatant. The lung distribution of ionic 141CeCl3 was similar, except a larger fraction was recovered in the supernatant (~15%) and to a lesser extent in the cell pellet (~20%). After 28 days, 88.3% and 74% of the instilled dose of nano-CeO2 and CeCl3, respectively, remained in the lungs.
The total amount of 141Ce from 141nano-CeO2 and 141CeCl3 retained in extrapulmonary tissues at 28 days was 0.9% and 6% of the total instilled dose, respectively. These were primarily detected in the liver and bone: 0.26 and 2.15% of nano-CeO2 and CeCl3, respectively, in liver; 0.32 and 2.50% of nano-CeO2 and CeCl3, respectively, in bone (see in Table 1 below). These data showed that translocation of instilled nano-CeO2 from the lungs was minimal. According to the authors, the higher translocation of ionic 141Ce was consistent with relatively faster lung clearance.
I.t. instillation of 141nano-CeO2 and 141CeCl3 resulted in significantly higher Ce tissue concentrations than after gavage exposure (see data in "nano-CeO2 - biodistribution in rats - oral - V2 2014Moli”) despite the fact that for nano-CeO2 Molina RM et al. administered a 5-fold lower dose than in gavage (1 vs. 5 mg/kg). Significantly higher Ce concentration was measured in lungs, bone marrow, bone, skin, testicles, kidneys, spleen, heart, liver, and gastrointestinal tract following instillation when compared to the results obtained following gavage administration.
Of note that the authors did not provide the data on control animals.
Konduru et al., 2015: The lung levels of 141Ce after a single IT instillation of radioactive uncoated CeO2 were evaluated in rats at 5 min, and 2, 7 and 28 days post-instillation. The authors observed that only ~8 % of the 141Ce from the CeO2 dose disappeared from the lungs during this period. By 28 days postinstillation, ~81 % CeO2 still remained in the lungs.
Low detectable fractions of radioactivity were found in the liver, bone/bone marrow, spleen and kidneys (<1 %) (see Table 2 below). - Details on excretion:
- Molina et al., 2014: Radioactive 141nano-CeO2 and 141CeCl3 were cleared slowly from the lungs with a half-life longer than 28 days, the last time point in the study. To estimate the clearance half-life, linear regression of the average lung 141Ce levels over time was performed. Correlation coefficients were 0.78 and 0.98 for 141nano-CeO2 and 141CeCl3, respectively. Extrapolated half-lives were ~140 days for nano-CeO2 and ~55 days for CeCl3. Clearance of 141nano-CeO2 was biphasic with a fast component during the first 2 days, followed by a slower phase seen through 28 days when 87.5% of the 141Ce remained in the lungs. Lung clearance of ionic 141CeCl3 was more linear. Still, only 26% of the CeCl3 dose was cleared at the end of observation period. The slow lung clearance correlated with very low dissolution of nano-CeO2 in simulated phagolysosomal fluid in vitro.
The elimination of 141Ce from either 141nano-CeO2 or 141CeCl3 was mostly via the faeces (~8.5 and ~24% of nano-CeO2 and CeCl3, respectively) and to a much lesser extent via the urine (~0.015 and ~0.13% of nano-CeO2 and CeCl3, respectively). The faecal and urinary elimination of 141Ce in rats instilled with 141CeCl3 was significantly higher than in the 141nano-CeO2 group.
Konduru et al., 2015: The elimination of 141Ce was mostly via the feces and to a much lesser extent via the urine. - Metabolites identified:
- not measured
- Conclusions:
- Nano-CeO2 was slowly cleared from the lungs but had minimal extrapulmonary accumulation.
=> Interpretation of results: high bioaccumulation and retention potential in lungs / low bioaccumulation potential in extrapulmonary organs based on study results - Executive summary:
Molina RM et al. (2014) and Konduru et al. (2015) investigated the bioavailability, tissue distribution, clearance and excretion of radioactive 141cerium (141Ce) after intratracheal (i.t.) instillation of neutron-activated 141cerium dioxide nanoparticles (141nano-CeO2) to male Wistar Han rats.
Commercial nano-CeO2, called NM-212, of 40 nm was used in the study of Molina et al., 2014 (provider: Mercator GmbH) and an in-house synthesized (of 32.9 nm) in the study of Konduru et al., 2015. Nano-CeO2 powder was neutron activated; the process generated the radioisotope 141Ce. The physico-characterisation was performed on non-radioactive CeO2, thus supposing that radioactive nano-CeO2 had the same physico-chemical properties as its stable counterpart. When suspended in distilled water, NM-212 displayed a hydrodynamic diameter of at least 207 nm and, for the in-house synthesized of, 136 nm; thus, the suspended nanoparticles agglomerated in aqueous suspension. The specific surface area of NM-212 and in-house synthesized nanoparticles were 27 and 28 m²/g, respectively. The surface charge of NM-212 was comprised between +-37 and +42 mV, depending on the physico-chemistry technique employed; but the suspended nano-CeO2 was always cationic in water and the suspension displayed a pH of 7.85. These roughly globular particles were crystalline (> 99% pure cerianite). The surface chemistry of nano-CeO2 NM 212 was composed of 79.9%, 17.7% O and 2.4% Ce, but total content of 0.7% organic contaminations, identified as ester + alkyl groups, was found on the particle surface as a very thin and homogeneous layer around the pure inorganic particles. Moreover, the oxidation degree of NM-212 surface was mainly as Ce(IV) but 14% were found as Ce(III). In addition, the nanoparticles were virtually insoluble under all conditions tested: for 24 h in DMEM with 10% foetal calf serum (FCS), for 28 days in phosphate-buffered saline (PBS, to simulate the surface of epithelium), 28 days in phagolysosomal simulant fluid (PSF, to simulate the phagolysosomes of macrophages), 1 day in 0.1N HCl (to simulate the stomach environment following oral intake), or 7days in a simulated intestinal fluid (fasted state, FaSSIF). Nano-CeO2 was also found to be photocatalytically active. The zeta potential of the in-house synthesized nano CeO2 was : +34,5 mv.
In both study, male rats (at least 5/gp) were exposed once to 0 (control) or 1 mg/kg of nano-CeO2 by i.t. instillation. Control rats were treated with sterile distilled water, being nano-CeO2 vehicle. All treated rats were sacrificed 5 min, 2, 7 and 28 days post-administration. Then, the following tissue samples were collected: blood, plasma, serum, lung, bone marrow, femoral bone, skin, brain, skeletal muscle, testicles, kidneys, spleen, heart, liver, stomach, small intestine, large intestine, and caecum. Moreover, 24-h samples of faeces and urine were collected at selected time points (0–24 hours, 2–3 days, 6–7 days, 9–10 days, 13–14 days, 20–21 days and 27–28 days post-gavage). The cerium (Ce) content in the samples was determined by measuring the radioactivity (141Ce) using a gamma counter. BALF parameters such as macrophages, lymphocytes, PMN or LDH were first measured in order to evaluate whether the concentration used for the instillation would induce lung injury.
The selected i.t. instillation dose of 1 mg/kg nano-CeO2 did not cause lung injury and inflammation. Sequential analyses of lungs over 28 days showed a high accumulation in the lungs (88.3% of the instilled dose after 28 days in the Molina's study and ~81 % in the Konduru's study) and slow lung clearance of 141nano-CeO2 (estimated half-life of ~140 days, Molina 's study). Translocation of nano-CeO2 to extrapulmonary organs was very low. About 0.86% (Molina's study) and < 1 % (Konduru's study) of instilled 141Ce was measured in selected extrapulmonary organs, such as liver, bone, caecum, large intestine (10 to 28 ng Ce/g tissue), stomach, bone, marrow, kidneys (5 to 10 ng/g), small intestine, spleen, skeletal muscle, heart (1 to 5 ng/g), or brain, blood, testicles, and skin (< 1 ng/g). The elimination of 141Ce from 141nano-CeO2 occurred mostly through faeces (~8.5 to ~19 % of the instilled dose) and to a much lesser extent through urine (~0.015 to 0,048 % of the instilled dose) as measured 28 days after instillation.
According to the authors, the slow lung clearance of nano-CeO2 may be due to poor solubility of CeO2, as shown by the absence of dissolution in simulant phagolysosomal fluid. This result was consistent with previous published data on insoluble or poorly soluble particles. Moreover, the significant association of nano-CeO2 with alveolar cells and lung interstitial tissues may have promoted its biopersistence.
Molina RM et al. concluded that, based on these results, low fractions of inhaled nano-CeO2 are expected to translocate to extrapulmonary tissues. Furthermore, the risk of extrapulmonary Ce tissue accumulation and potential toxicity is expected to be low when nano-CeO2 is inhaled. Further, the authors said that the lung clearance observed in both studies that used 2 different nano CeO2 were similar.
- Endpoint:
- basic toxicokinetics in vivo
- Type of information:
- experimental study
- Adequacy of study:
- supporting study
- Study period:
- Not applicable
- Reliability:
- 3 (not reliable)
- Rationale for reliability incl. deficiencies:
- other: :
- Remarks:
- the statistical analyses were insufficiently documented and some methods and results were not clearly described, which made it difficult to understand (e.g., no controls animals). Moreover, the physico-chemical characterisation was performed on non-radioactive nano-CeO2 instead of the radioactive nanoparticles which were the material administered to animals.
- Reason / purpose for cross-reference:
- reference to same study
- Objective of study:
- distribution
- excretion
- Qualifier:
- no guideline followed
- Principles of method if other than guideline:
- - Single intratracheal instillation of a suspension of nanometric cerium dioxide (nano-CeO2) in rats; sacrifice of animals 6 h, 24 h, 7 d and 28 d after administration
- Pulmonary retention, elimination half-life, extrapulmonary translocation and excretion: radiotracer technique (i.e., radioactive nano-CeO2) - GLP compliance:
- not specified
- Remarks:
- The GLP status was not specified in the article.
- Radiolabelling:
- yes
- Species:
- rat
- Strain:
- Wistar
- Sex:
- male
- Details on test animals or test system and environmental conditions:
- TEST ANIMALS
- Source: Experimental Animal Center, Peking University (China)
- Age at study initiation: No data available
- Weight at study initiation: 190-210 g
- Fasting period: Deprivation of food at the beginning of intratracheal exposure and until 6 h after instillation (access to water remained available)
- Housing: Individually in stainless steel cages
- Individual metabolism cages: No data available
- Diet: Ad libitum (commercial pellet diet)
- Water: Ad libitum (deionized water)
- Acclimation period: One week
ENVIRONMENTAL CONDITIONS
- Temperature: 20 ± 2°C
- Humidity: 50-70% relative humidity
- Air changes: No data available
- Photoperiod: 12 hrs dark / 12 hrs light
IN-LIFE DATES: No data available - Route of administration:
- intratracheal
- Vehicle:
- other: Milli-Q ultrapure water
- Details on exposure:
- Four groups of rats were exposed to nano-CeO2 via intratracheal instillation. After the anaesthetized rat was restrained on a surgical borad at a ~60° angle, the trachea was exposed surgically on the ventral side of the neck. A needle was inserted through the tracheal wall into the lumen just below the larynx, and 0.1 mL of the nano-CeO2 suspension was injected into the trachea. The animal was maintained in an upright position for 5 min after instillation to allow the fluid to drain into the respiratory tree. Then, the incision was sutured and rats were kept in a 30°C incubator until it regained consciousness.
- Duration and frequency of treatment / exposure:
- A single exposure (sacrifice 6 h, 24 h, 7 d and 28 d after intratracheal administration)
- Dose / conc.:
- 2 other: mg/mL
- Remarks:
- 0.1 mL of a suspension of 2 mg/mL
basis: nominal nano-CeO2 conc. - No. of animals per sex per dose / concentration:
- 6
- Control animals:
- no
- Details on dosing and sampling:
- PHARMACOKINETIC STUDY (Absorption, distribution, excretion)
Fasted rats were sacrificed under anaesthesia at 6 h, 24 h, 7 d, and 28 d after intratracheal instillation and blood was collected by heart puncture. After saline perfusion to remove the residual blood, the lung, heart, liver, spleen, kidney, stomach, intestine, testicle, brain, left femur, skeletal muscle, and urine of each rat were collected. Blood samples were collected at 10 min, 30, min, 1 h, 2 h, 4 h, 24 h, 7 d and 28 d post-instillation from the caudal vein of exposed rats. The daily faeces and urine of rats killed at 28 d post-instillation were collected individually.
RADIOACTIVITY DETERMINATION
Nano-CeO2 biodistribution was determined by measuring the activities of 141Ce in samples using a photon detector with a relative detection efficiency of 30%. The content of nano-CeO2 was calculated by comparing the radioactivity in the sample to the reference standard. The data were expressed as the content in tissues or the percentage of the given dose. The pharmacokinetics of intratracheally instilled nano-CeO2 in the lung was evaluated using a first-order kinetics model. - Statistics:
- No data available
- Preliminary studies:
- No data available
- Details on absorption:
- After 200 µg (0.1 mL of a suspension of 2 mg/mL) of nano-CeO2 was introduced by intratracheal instillation, the deposited nano-CeO2 was cleared slowly from the host lung. Nearly 80% of the instilled nano-CeO2 was deposited in the lung regions at 24 h post-instillation (see in Table 2 below). At day 28 post-exposure, 63.9 ± 8.2% of the administered dose still remained in the lung.
- Details on distribution in tissues:
- Nano-CeO2 content in blood slightly increased within 10 min after instillation and the transported particles could be found in extrapulmonary tissues suggesting that intratracheally instilled nano-CeO2 could penetrate through the alveolar-capillary barrier into the systemic circulation. Small but detectable extrapulmonary translocation and redistribution of nano-CeO2 had taken place in an organ-specific and selective manner: testicles, brain < 5 x10E-5 µg/g ≤ muscle, kidney < 1 x 10E-4 µg/g bone ≤ spleen, blood, liver, heart ≤ 5 x 10E-4 µg/g < bone ≤ 0.001 µg/g < stomach, intestine ≤ 0.5 µg/g wet tissue at 6 h post-exposure (see in Table 2 below). Though the particle fractions in liver and spleen demonstrated a tendency towards time-dependent increase and that nano-CeO2 contents increased by two orders in both organs during the test period, such a trend was not apparent in the other organs such as stomach or heart. At day 28 post-instillation, about 0.1 and 0.01% of the administered dose were deposited in liver and spleen, respectively. According to the authors, the amounts of nano-CeO2 in blood and bone were at a level similar to those in liver or spleen. Nano-CeO2 contents remained at a low level in other tissues (~ 0.00001 to 0.001% for brain, testicles, heart, stomach, and kidney).
- Details on excretion:
- The initial clearance rate was calculated to be 1.03 µg/d and the elimination half-life was of 103 days. Apparently, most of nano-CeO2 lost from the lung could be found in faeces and little could be detected in urine samples (below 1 x 10E-7 µg/g at each time point; data not shown for urine measurements).
During the first 3 days, nano-CeO2 content in faeces decreased sharply. The major part of nano-CeO2 in the excretion might be those directly returned to the larynx or removed via mucociliary clearance (estimated at 23 % in this study). At days 4-7 post-exposure, the clearance rate ranged from 0.98 to 1.00 µg/d and the daily content of nano-CeO2 in faeces was more than 0.8 µg/d, suggesting that eliminated nano-CeO2 from the lung was mainly cleared via the alveolar macrophage (AM)-mediated route and then excreted during this period. However, from days 8 to 28 post-exposure, the calculated clearance rate was ~0.8-1.0 µg/d, while, from day 10 post-instillation on, daily nano-CeO2 content in faeces, representing AM-mediated nano-CeO2 transport from the lung to larynx, was below 0.4 µg, and at day 28 post-exposure nano-CeO2 content in faeces was 0.10 ± 0.07 µg. The comparison between calculated clearance rate and observed excretion rate suggested that only about 1/8-1/3 of the daily elimination of nano-CeO2 was cleared via AM-mediated route with subsequent transport from lung to larynx.
He X et al. noted that the total increase in nano-CeO2 in the collected samples and faeces could not equal the amount of nano-CeO2 cleared from the lung, thus they speculated that some of the particles were retained in the rat carcass, especially in the lymph nodes. - Metabolites identified:
- not measured
- Bioaccessibility (or Bioavailability) testing results:
- No data available
- Conclusions:
- Intratracheally instilled nano-CeO2 were mainly accumulated in the lungs and could penetrate through the alveolar-capillary barrier into the systemic circulation within 10 min. Penetration of nano-CeO2 to body circulations increased while alveolar macrophage-mediated nanoparticle transport from lung to gastro-intestinal tract (allowing excretion) declined at the end of the test period. A small but detectable extrapulmonary translocation and redistribution of nanoparticles occurred in an organ-specific and selective manner: liver and spleen were mainly targeted.
=> Interpretation of results: pulmonary bioaccumulation potential of instilled nano-CeO2 / low bioaccumulation potential in other organs regardless of the route of administration - Executive summary:
He X et al. (2010) employed the radiotracer technique to study the pulmonary deposition and the translocation of nanometric cerium dioxide (nano-CeO2) to secondary target organs into intratracheally instilled rats.
Radiolabelled nano-CeO2 was used in this study. The nanoparticles were synthesised from radioactive Ce(NO3)3 using a precipitation method. However, as the authors assumed that the radiolabelled and non-labelled nanoparticles had the same physico-chemical properties, only the non-radiolabelled nano-CeO2 were characterised. The insoluble and crystalline nano-CeO2 had a primary size of 6.6 nm, a hydrodynamic size of 12.8 nm, a specific surface area of 86.85 m²/g and a zeta potential of +30.1 mV. He X et al. also tested the stability of non-radioactive nano-CeO2 suspended in various media. The results showed that nano-CeO2 agglomerated in all media (e.g. ultrapure water, or physiological saline solution), but adding serum or albumin reduced, or even prevented, nano-CeO2 agglomeration.
Male Wistar rats (6/group) were intratracheally exposed to 0.1 mL of a nano-CeO2 suspension at 2 mg/mL in Milli-Q ultrapure water (sonicated for 15 min before using), and then sacrificed at 6 h, 24 h, 7 d, and 28 d after exposure. Cerium content, based on radioactivity measurements of 141Ce, was quantified in blood, lungs, heart, liver, kidney, spleen, stomach, intestine, testicle, brain, left femur, skeletal muscle, faeces and urine using a photon detector.
The intratracheal study showed that 24 h after exposure nearly 80% of the instilled nano-CeO2 was deposited in the lung regions and 63.9 ± 8.2% of the particles remained in the lung by 28 days post-exposure. The deposited nano-CeO2 was cleared slowly from the host lung. The initial clearance rate was calculated to be 1.03 µg/d and the elimination half-life was of 103 days. Nano-CeO2 content in blood slightly increased within 10 min after instillation (< 10E-6 % from 6 h to 28 days post-i.t.) and the transported particles could be found in extrapulmonary tissues suggesting that intratracheally instilled nano-CeO2 could penetrate through the alveolar-capillary barrier into the systemic circulation and accumulate in the following extrapulmonary organs: liver (0.0005% and 0.1% at 6 h and 28 days post-i.t., respectively), spleen (≤ 0.0001% and 0.01% at 6 h and 28 days post-i.t., respectively), and kidney (0.00005% and ≤ 0.005% at 6 h and 28 days post-i.t., respectively). Though the particle fractions in liver and spleen demonstrated a tendency towards time-dependent increase and that nano-CeO2 contents increased by two orders in both organs during the test period, such a trend was not apparent in the other organs such as stomach or heart. Nano-CeO2 contents remained at a low level in other tissues from 6 h to 28 d post-i.t. (< 10E-6 % to ≤ 0.001% for brain, testicles, heart, or stomach). At the end of the test period, only 1/8-1/3 of the daily elimination of nano-CeO2 from the lung was cleared via the gastrointestinal tract, suggesting that phagocytosis by alveolar macrophages (AMs) with subsequent removal towards the larynx was no longer the predominant route for the elimination of nano-CeO2 from the lung. Really low level (below 1 x 10E-7 µg/g at each time point) was found in urine.
Even though the results described in this publication seemed in adequacy with previous studies on nano-CeO2 biodistribution, it was difficult to assess the relevance of these data given the lack of statistics, some unclear descriptions of the methods, and the absence of control animals. The latter point would have been interesting since some data on Ce content in blood or tissues were so low that this could be the result of a contamination or background level.
- Endpoint:
- dermal absorption in vitro / ex vivo
- Type of information:
- experimental study
- Adequacy of study:
- key study
- Study period:
- 2019
- Reliability:
- 2 (reliable with restrictions)
- Rationale for reliability incl. deficiencies:
- guideline study with acceptable restrictions
- Remarks:
- The test substance was not fully characterized for nano characterisation parameters. The methods and results are reported quite briefly.
- Qualifier:
- according to guideline
- Guideline:
- OECD Guideline 428 (Skin Absorption: In Vitro Method)
- Qualifier:
- according to guideline
- Guideline:
- other: Franz method
- Version / remarks:
- As published in: Guth, K.; Schäfer-Korting, M.; Fabian, E.; Landsiedel, R.; van Ravenzwaay, B. Suitability of skin integrity tests for dermal absorption studies in vitro. Toxicol. In Vitro 2015, 29, 113–123.
- Qualifier:
- according to guideline
- Guideline:
- other: EDETOX protocols
- Version / remarks:
- As published in: Sandt, J.J.; Burgsteden, J.A.; Cage, S.; Carmichael, P.L.; Dick, I.; Kenyon, S.; Korinth, G.; Larese, F.;
Limasset, J.C.; Maas, W.J.; et al. In vitro predictions of skin absorption of caeine, testosterone, and benzoic acid: A multi-centre comparison study. Regul. Toxicol. Pharmacol. 2004, 39, 271–281. - GLP compliance:
- not specified
- Remarks:
- The GLP status was not specified in the article.
- Radiolabelling:
- no
- Species:
- other: Human
- Strain:
- other: not applicable
- Sex:
- female
- Details on test animals or test system and environmental conditions:
- Not appropriate. See information reported in the section "Details on study design".
- Type of coverage:
- other: no applicable
- Vehicle:
- other: synthetic sweat
- Duration of exposure:
- 24 hoours
- Doses:
- 0,6 mg cerium dioxide/cm2 corresponding to 0,220 ml of the donor solution (synthetic sweat).
- No. of animals per group:
- not applicable
- Details on in vitro test system (if applicable):
- SKIN PREPARATION
- Source of skin: Human abdominal full-thickness skin was obtained as surgical waste from two female donors aged 45–65 years. Others donors were men and women with an age range of 45–71 years.
- Ethical approval if human skin: yes. The study was approved by the Trieste Hospital Ethical Committee n° 236/2007.
- Type of skin: Human abdominal full-thickness skin
- Preparative technique: After the skin excision, subcutaneous fat was removed with a scalpel blade and hair was shaved from the epidermis. Two different experiments were conducted using intact and damaged skin.
- Thickness of skin (in mm): approximately 1 mm.
- Membrane integrity check: Yes. Skin integrity was assessed using the transepidermal water loss (TEWL) method as described by Guth et al. (2015). Cells with a value of >10 g/m2/h were considered to be damaged and rejected.
- Storage conditions: Skin samples were stored at -25 °C for a period up to, but not exceeding, four months. It has been demonstrated that this procedure does not damage skin barrier properties. On the day of the experiment, skin samples were defrosted in a physiological solution at room temperature for a 30-min period and 2 x 2 cm2 pieces were cut from each skin specimen and mounted separately on the diffusion cells.
- Justification of species, anatomical site and preparative technique: no data
PRINCIPLES OF ASSAY
- Diffusion cell: Franz cell
- Receptor fluid: physiological solution. The physiological solution used as the receptor phase was prepared by dissolving 2.38 g of Na2HPO4, 0.19 g of KH2PO4, and 9 g of NaCl into 1 L of Milli-Q water (final pH 7.35). The salt concentration in the receiving fluid was approximately the same as that found in blood.
- Solubility od test substance in receptor fluid: No data.
- Static system: Yes
- Flow-through system:
- Test temperature: 32 ° C
- Humidity: no data
- Occlusion: no
- Reference substance(s): no
- Other
Donor fluid: synthetic sweat solution consisting of 0.5% sodium chloride, 0.1% urea, and 0.1% lactic acid in Milli-Q water, and pH was adjusted to 4.5 using a concentrated ammonia solution. The authors indicated that the cerium concentration in the donor phase, which was collected at the beginning of the test after the removal of the NPs by ultrafiltration, was less than 0.05% of the starting suspensions and did not change at the end of the experiments.
- Signs and symptoms of toxicity:
- not examined
- Remarks:
- in vitro study
- Dermal irritation:
- not examined
- Remarks:
- in vitro study
- Absorption in different matrices:
- - Receptor fluid, receptor chamber, donor chamber (in vitro test system):
The cerium concentration in the donor phase, which was collected at the beginning of the test after the removal of the NPs by ultrafiltration, was less than 0.05% of the starting suspensions and did not change at the end of the experiments.
In the experimental condition, the used Ce did not permeate the intact skin since the concentration in the receiving phase was the same as that of the blank cells (2.0 +/- 0.4 ng/cm2) after 24 hours. However, it reached 3.3 +/- 0.7 ng/ cm2 for damaged skin (p = 0.008).
The amount of Ce (µg/cm2) inside whole skin was 3.64 +/- 0.15 for intact skin, 7.07 +/- 0.78 for damaged skin, and 0.19 +/- 0.06 for blank cells (mean +/- SD). The Ce content was higher in dermal layers of damaged skin compared to intact skin (2.93 +/- 0.71 µg/cm2 and 0.39 +/- 0.16 µg/cm2, respectively).
Microscopic analysis confirmed the low penetration and permeation of CeO2 NPs since only a small signal of Ce was revealed in the epidermis of an intact skin sample but NPs were not
visualized by TEM investigation.
- Skin preparation (in vitro test system): see above in "Details on in vitro test system" and "Any other information on materials and methods incl tables"
- Stratum corneum (in vitro test system): not applicable. - Key result
- Time point:
- 24 h
- Dose:
- vehicle (synthetic sweat)
- Parameter:
- amount
- Remarks:
- Amount of Ce that penetrate into the whole skin.
- Absorption:
- ca. 0.19 other: µg/cm2
- Remarks on result:
- other: For blank cells
- Key result
- Time point:
- 24 h
- Dose:
- 0,6 mg CeO2 /cm2
- Parameter:
- amount
- Remarks:
- Amount of Ce that penetrate into the whole skin.
- Absorption:
- ca. 3.64 other: µg/cm2
- Remarks on result:
- other: for intact skin.
- Key result
- Time point:
- 24 h
- Dose:
- 0,6 mg CeO2/cm2
- Parameter:
- amount
- Remarks:
- Amount of Ce that penetrate into the whole skin.
- Absorption:
- ca. 7.07 other: µg/cm2
- Remarks on result:
- other: For damaged skin
- Key result
- Time point:
- 24 h
- Dose:
- vehicle (synthetic sweat)
- Parameter:
- amount
- Remarks:
- Amount of Ce that penetrate into the dermis layer.
- Absorption:
- > 0 - <= 0.1 other: µg/cm2
- Remarks on result:
- other: For blank cells
- Key result
- Time point:
- 24 h
- Dose:
- 0,6 mg CeO2/cm2
- Parameter:
- amount
- Remarks:
- Amount of Ce that penetrate into the dermis layer.
- Absorption:
- ca. 0.39 other: µg/cm2
- Remarks on result:
- other: For intact skin
- Key result
- Time point:
- 24 h
- Dose:
- 0,6 mg CeO2/cm2
- Parameter:
- amount
- Remarks:
- Amount of Ce that penetrate into the dermis layer.
- Absorption:
- ca. 2.93 other: µg/cm2
- Remarks on result:
- other: For damaged skin.
- Key result
- Time point:
- 24 h
- Dose:
- vehicle (synthetic sweat)
- Parameter:
- amount
- Remarks:
- Ce concentration in the receiving solution
- Absorption:
- ca. 2 other: ng/cm2
- Remarks on result:
- other: Blank Cells
- Key result
- Time point:
- 24 h
- Dose:
- 0,6 mg CeO2/cm2
- Parameter:
- amount
- Remarks:
- Ce concentration in the receiving solution.
- Absorption:
- ca. 2 other: ng/cm2
- Remarks on result:
- other: For intact skin.
- Key result
- Time point:
- 24 h
- Dose:
- 0,6 mg CeO2/cm2
- Parameter:
- amount
- Remarks:
- Ce concentration in the receiving solution
- Absorption:
- ca. 3.3 other: ng/cm2
- Remarks on result:
- other: For damages skin.
- Conclusions:
- The authors concluded that their data showed a very low dermal absorption and transdermal permeation of cerium dioxide nanoparticles.
- Executive summary:
Mauro M. et al, (2019) performed an in vitro investigation of the permeation of in-house syntesized 17-nm CeO2 NPs dispersed in synthetic sweat (1 g/L) using excised human skin on Franz cells. The study was performed according to OECD guideline n° 428, but no information on the GLP status was indicated in the article. Thus, the study was scored as validity 2 according to Klimisch criteria. Experiments were performed using intact and needle-abraded skin, separately.
In intact and damages skin permeation experiments at time 0, the exposure chambers of three Franz dffusion cells were filled with 0.220 mL of the donor solution, corresponding to an amount of CeO2 of 0.6 mg/cm2. The experiment was repeated twice. After the integrity test, the receiving solutions and the skin pieces were removed and analysed for CeO2 content.
After 24 hours, the average amount of Ce into intact and damaged whole skin samples was 3.64 +/- 0.15 and 7.07 +/- 0.78 µg/cm2, respectively. Ce concentration in the receiving solution was 2.0 +/-0.4 (as in the blank cells) and 3.3 +/- 0.7 ng/cm2 after 24 h. The Ce content was higher in dermal layers of damaged skin compared to intact skin (2.93 +/- 0.71 µg/cm2 and 0.39 +/- 0.16 µg/cm2, respectively).
The authors concluded that CeO2 NPs could not permeate intact skin, but this permeation was possible—if at a low level—using an abraded skin protocol. A low amount of cerium was present in intact and damaged skin samples after prolonged exposure (24 h) to CeO2NPs. They further suggested that this behavior is probably due to the very low ionization of these metal oxides in synthetic sweat, resulting in a small concentration of free metal ions in the donor phase being able to cross over the physiological barriers. The NPs’ capability of permeating the dermal layers is lower with respect to the free ions, and depends on their physicochemical characteristics.
- Endpoint:
- basic toxicokinetics in vivo
- Type of information:
- experimental study
- Adequacy of study:
- key study
- Study period:
- 2013 - 2020
- Reliability:
- 1 (reliable without restriction)
- Rationale for reliability incl. deficiencies:
- guideline study
- Reason / purpose for cross-reference:
- reference to other study
- Objective of study:
- absorption
- distribution
- Qualifier:
- according to guideline
- Guideline:
- other: OECD test guideline 453
- Version / remarks:
- An organ burden study with nano CeO2 was performed as part of a carcinogenicity study performed according to OECD test guideline no. 453.
- Principles of method if other than guideline:
- The CeO2 burden for lung, lung-associated lymph nodes, and major non-pulmonary organs, blood, and feces, was determined in a chronic whole-body inhalation study in female Wistar rats undertaken according to OECD TG453. Female rats were whole body exposed to nano CeO2 NM-212, 6 h per day for 5 days per week for a 104 weeks with the following concentrations: 0, 0.1, 0.3, 1.0, and 3.0 mg/m3. Then, animals were sacrificed after 3, 12, 24 months. Different spectroscopy methods (ICP-MS, ion-beam microscopy) were used for the quantification of organ burden and for visualization of NP distribution patterns in tissues.
- GLP compliance:
- yes
- Radiolabelling:
- no
- Species:
- rat
- Strain:
- Wistar
- Sex:
- female
- Details on test animals or test system and environmental conditions:
- TEST ANIMALS
- Source: Charles River Laboratories (Sulzfeld, Germany).
- Age at study initiation: 7 weeks of age
- Weight at study initiation: not specified in this article
- Housing: The animals were maintained in groups of up to five animals in polysulfon cages (TECNIPLAST, Germany) with access to wooden gnawing blocks.
- Diet (e.g. ad libitum): ad libitum. GLP certified feed (Kliba laboratory diet, Provimi Kliba SA, Kaiseraugst, Basel, Switzerland).
- Water (e.g. ad libitum): ad libitum
- Acclimation period: not specified in this article
ENVIRONMENTAL CONDITIONS
- Temperature (°C): not specified in this article
- Humidity (%): not specified in this article
- Air changes (per hr): not specified in this article
- Photoperiod (hrs dark / hrs light):not specified in this article
IN-LIFE DATES: From: To: not specified in this article - Route of administration:
- inhalation: aerosol
- Vehicle:
- unchanged (no vehicle)
- Details on exposure:
- For details, see Keller et al. (2015) in IUCLID scetion 7.5.2
- Duration and frequency of treatment / exposure:
- The animals were exposed to CeO2 aerosols for a total of 104 weeks (2 years). Exposure to CeO2 NPs was 5 days a week for 6 h per day.
- Dose / conc.:
- 0 mg/m³ air (nominal)
- Remarks:
- Control
- Dose / conc.:
- 0.1 mg/m³ air (nominal)
- Remarks:
- Measured concentrations : 0,1 +/- 0,1 mg/m3 +/- SD
- Dose / conc.:
- 0.3 mg/m³ air (nominal)
- Remarks:
- Measured concentrations : 0,3 +/- 0,1 mg/m3 +/- SD
- Dose / conc.:
- 1 mg/m³ air (nominal)
- Remarks:
- Measured concentrations : 1,0 +/- 0,1 mg/m3 +/- SD
- Dose / conc.:
- 3 mg/m³ air (nominal)
- Remarks:
- Measured concentrations : 3,0 +/- 0,4 mg/m3 +/- SD
- No. of animals per sex per dose / concentration:
- For the 3 and 12 months’ time point analyses, three animals per dose group were sacrificed for burden analyses. For the 24 months’ time point analyses four animals per dose group were used for this study.
- Control animals:
- yes, concurrent vehicle
- Positive control reference chemical:
- no
- Details on study design:
- - Dose selection rationale: The concentrations used in the 2-year study were chosen on the basis of results from a preliminary 28 day study (Keller et al., 2014) summarized in IUCLID Section 7.5.2.
- Exposure conditions during the study: The animals were exposed whilst being in wire cages located in a stainless-steel whole-body inhalation chamber (V=2.8m3 or V=1.4m3). The aerosols entered the inhalation chambers with the supply air and were removed by an exhaust air system with 20 air changes per hour. Characterization, generation, and monitoring of aerosols, animal whole-body exposure, and all clinical pathology were performed at BASF SE Ludwigshafen, Germany. For more information, see Keller (2015) as summarized in IUCLID scetion 7.5.2. - Details on dosing and sampling:
- TOXICOKINETIC / PHARMACOKINETIC STUDY
Organ burden, distribution and localisation of nano CeO2 were analysed in this study:
- Tissues and body fluids sampled : brain, olfactory bulbs, lungs, kidneys, liver, heart, spleen, and small intestine (jejunum). Feces were taken directly from the intestine of the sacrificed animals of the appropriate dose group. Additionally, bone, bone marrow, and blood samples were taken at the same time points. For the lymph nodes, a distinction was made between tracheobronchial, mediastinal, and mesenteric lymph nodes. The tracheobronchial lymph nodes were analyzed after 3, 12, and 24 months of exposure, while the mesenteric lymph nodes were removed at 12 and 24 month time points and the mediastinal at 24 months.
- Time and frequency of sampling: Animals were sacrificed at 3, 12, and 24 months.
- Other: For the 3 and 12 months’ time point, three animals per dose group were sacrificed, for the 24 months’ time point four animals per dose group were used. - Statistics:
- Data from CeO2 contents of each test group from different time points were used in a two-way ANOVA for calculating dependencies between organ burden and exposure concentration, as well as between organ burden and time. Results were statistically significant at p ≤ 0.05. Dunnett’s test was used to compare CeO2 tissue contents between the control group and each test group. For calculations the software R3.03 was used.
Outliers for each organ within each test group were identified using the Grubbs test.(Grubbs, 1950). Deposition of CeO2 of each organ and test group was illustrated applying software REGRESSA.
Results of tissue-related CeO2 contents are presented as mean value ± standard deviation (SD) for liver, kidneys and spleen (n = 3 each) on the last day of exposure (except spleen dose group #1, n = 2). All other data points reflect the CeO2 values of one organ and time point per animal.
The measured elemental tissue cerium contents were converted to CeO2, because CeO2 NPs were previously assumed to remain nanoparticles (NPs) even after distribution and deposition. - Preliminary studies:
- Not applicable
- Details on absorption:
- > Lung burden:
During the 2-year inhalation study, the lung burden was evaluated after 3, 12, and 24 months. After 24 months of exposure, the maximum CeO2 lung burden (5.88mg CeO2/lung) with a mean value of 4.4 ± 1.0 mg/lung was found associated with the highest aerosol concentration of Group 4. The lung burden (in µg/lung +/- SD) of each test groups after 3, 12 and 24 month of exposure were as follow (see also Table 1 below in "Any other information on results incl. tables):
Control group: 1.23 ± 0.63, 1.56 ± 0.63, 1.35 ± 036 µg/lung at 3, 12 and 24 months of exposure, respectively.
0,1 mg/m3 group (Group # 1): 19.92 ± 2.13, 49.22 ± 11.46, 78.02 ± 34.91 µg/lung at 3, 12 and 24 months of exposure, respectively.
0,3 mg/m3 group (Group # 2): 48.47 ± 5.65, 227.83 ± 27.71, 348.4 ± 54.25 µg/lung at 3, 12 and 24 months of exposure, respectively.
1 mg/m3 group (Group # 3): 258.14 ± 24.94, 924.36 ± 99.96, 1450.07 ± 275.03 µg/lung at 3, 12 and 24 months of exposure, respectively.
3 mg/m3 group (Group # 4): 772.03 ± 126.45, 2603.02 ± 337, 4411.94 ± 1038.55 µg/lung at 3, 12 and 24 months of exposure, respectively.
For all aerosol concentrations tested (Groups #1 to #4), a linear increase in lung burden was observed over time (R2 values ranging from 0.96 to 0.99). No difference in the curve shape between the lowest and the highest dose group was observed. The highest dose group of 3 mg/m3 (m =172) showed approximately a threefold higher slope than the next lower exposure concentration (dose Group #3; m =56). The same relationship was found between the other dose groups, except dose Group #1 with a slightly lower m value as expected (m =3). This indicates a proportional relationship for exposure dose versus time and amongst, at least, for the aerosol exposure Groups #2–#4. The predicted and measured lung burden was found to match reasonably well with however, some underestimation of measured lung burden values, especially after the 12-month time point, according to the authors.
A retention half-time of 164 days was calculated for dose Group #3, which was higher than expected. The calculated retention half-time for Group #4 is 200, which would mean the overload condition of the lung is reached (see also Table 1 in "Any other information on results incl. tables). Based on the aerosol characteristics measured during NP exposure, a prediction of the deposited alveolar fraction was generated using the MPPD model. For the four aerosol concentrations (#1–#4), an alveolar deposition fraction of around 12% was determined (see also Table 1 in "Any other information on results incl. tables). - Details on distribution in tissues:
- > Lymph nodes burden:
As lymph nodes play a key role in lung clearance, three different lymph node regions were investigated to measure their CeO2 load. The lung associated lymph nodes (LALN), comprising the tracheobronchial plus the mediastinal lymph nodes and the mesenteric lymph nodes were analyzed individually for all 4 dose groups (see Table 3 in "Any other information on results incl. Tables"). The NP contents of LALN reached a level of 2400 ± 570 µg/organ CeO2 in Group # 4 at 24 months of exposure. These results show that the LALN carry the highest CeO2 burden from all investigated organs besides the lung, for all dose groups at all studied time points, whereas CeO2 values of the mesenteric lymph nodes are more than 10 000 times lower (<80 ng of total CeO2 in all mesenteric lymph nodes collected).
Visaulisation of CeO2 NP in lung tissue samples using ToF-SIMS, ligt microscopy and mass spectrometry showed NP agglomerates within the lung sections of the animals group # 4 at 24-month time point. Tissue sections of the 2-year study revealed an inhomogeneity throughout the whole lung. NP concentrations in different lung tissue areas varied rather widely. The particles were found mainly in macrophages. In addition, the alveolar septum contained some smaller NP agglomerates. Large NP agglomerates are concentrated in the bronchus-associated lymphoid tissue (BALT) with smaller agglomerates distributed throughout the remaining lung tissue.
Very large agglomerates (category 10: >200 mm2) of CeO2 NP or a fraction of very small agglomerates (category 1: 1–1.49 mm2) were found in the test group # 4 (3.0 mg/m3) after 24 months of exposure. The small and medium-sized agglomerates are either significantly less abundant or completely absent. In the the next lower dose (group # 3, 1 mg/m3), after 24 months, all observed NP agglomerates were smaller than 200 mm2. The highest number of agglomerates was observed in category 6 (6.1–12.17 mm2).
Extrapulmonary distribution (see Table 3 in "Any other information on results incl. Tables"):
The absolute CeO2 concentrations in the control groups for each time point (3, 12 and 24 months of exposure) were very low. The average values ranged from 0.001 to 0.04 ng/mg for tissue and from 0.0001 mg/mg to 0.02 mg/mg for feces.
The cerium blood concentrations for all dose groups were below or close to the limit of detection (0.04 mg/ mL) and thus negligible.
Generally, the highest extra-pulmonary organ burden was detected in Group #4 and was lower in Groups 3, 2, and 1 (see also Table 3 in "Any other information on results incl. tables). Besides the LALN, CeO2 burden was highest in liver (~12 µg CeO2/organ, in group 4 at time point 24 months) with the following ranking liver > kidneys, spleen >>> heart > brain > olfactory bulb after 24 months. The ratios of liver to lung burdens are low with a peak at the 12-month time point followed by a leveling off or reduction for all investigated exposure concentrations. For brain and heart no significant changes in CeO2 organ burden compared to control levels were detected after 24 months of exposure. This holds also true for the Ce-concentrations measured in blood. Relatively high amounts of Ce was found in femur bones (~1800 +/- 400 ng/g) and bone marrow (350 +/- 110 ng/g) in animals of group # 4 after 24 month of exposure in comparison to all other extrapulmonary organs, except LALNs. The burden of the skeleton in the same animal group was calculated to be 44.9 µg/skeleton (calculated from the CeO2 concentration in femur tissue and the assumption of a homogenous distribution and a skeleton weight corresponding to 6 % of the body weight of the rat). According to the authors, under these assumptions, around 1.1% of the lung burden (4412 µg/lung) would be retained in the skeleton for the group #4. In comparison, 1.2% of the lung burden was found in all investigated extra-pulmonary organs excluding lymph nodes and skeleton. - Details on excretion:
- From the results reported in the section "details on distribution in tissues" and in Table 3 in "Any other information on results incl. tables, the authors concluded that, of all investigated organs excluding the lung, lymph nodes in close proximity of the lung carry the highest NP burden resulting from particles deposited in the alveolar region indicating the primary excretory route.
A clearance via fecal excretion was also observed since an increase of CeO2 concentrations (as µg/g of feces) was observed in all treated groups between 3 and 12 months followed by a decrease between month 12 and 24 (see Table 3 in "Any other information on results incl. tables). When calculated by day, considereing 4 g/day of feces, fecal excretion reached 64 to 180 µg/day in the group #4 during the study. - Conclusions:
- After exposure by inhalation to 0.1, 0,3, 1 and 3 mgm3 nano CeO2 for 24 month in an OECD 453 study, the CeO2 burden in the organs analysed was found with the following ranking: lungs > lymph node > hard bone > liver > bone marrow > kidneys, spleen >>> heart > brain > olfactory bulb. According to the authors, estimated alveolar deposition fraction was found to be around 12 % and 1.2% of the lung burden was found in all investigated extra-pulmonary organs excluding lymph nodes and skeleton and around 1.1% of the lung burden would be retained in the skeleton for the group #4.
- Executive summary:
The CeO2 organ burden, distribution and localisation was determined in a chronic whole-body inhalation study in female Wistar rats performed according to OECD TG 453 and in compliance with GLP. Groups of female rats were whole body exposed to nano CeO2 NM-212, 6 h per day for 5 days per week for a 104 weeks with the following concentrations: 0 (control- air), 0.1, 0.3, 1.0, and 3.0 mg/m3. The CeO2 burden was measured in lung, lung-associated lymph nodes, brain, olfactory bulbs, kidneys, liver, heart, spleen, small intestine (jejunum), bone (femur), bone marrow, blood and in feces in 3 to 4 animals/groups after 3, 12, 24 months of exposure. Quantification of CeO2 organ burden and visualization of nanoparticule distribution in tissues were performed using ICP-MS and ion-beam microscopy. Experimentally quantified lung burdens were compared with predictions of the deposited alveolar burden using the software an MPPD model.
The lung and the lung associated lumph nodes (LALNs) were found to contain the highest burden of CeO2. After 24 months of exposure, the mean CeO2 lung burden was found to be 1 ± 0,3, 78 ± 35, 348 ± 54, 1450 ± 275 and 4412 ± 1030 µg/lung in groups exposed to 0, 0.1, 0.3, 1.0, and 3.0 mg/m3, respectively. For all aerosol concentrations tested, a linear increase in lung burden was observed over time. The alveolar deposition fraction was estimated using MMPD model around 12% for all exposed groups. The nanoCeO2 contents of the lung associated lymph nodes reach of level of 2372 ± 560 µg CeO2/organ in the high dose concentration group after 24 months of exposure whereas the CeO2 content in mesenteric lymph nodes in the same animal group was = 73 +/- 20 ng/organ. Inhomogenous nanoparticle agglomerates were foung throughout the whole lung. Large NP agglomerates (several up to > 200 mm²) are concentrated in the bronchus-associated lymphoid tissue (BALT) with smaller agglomerates distributed throughout the remaining lung tissue. The particles were found mainly in macrophages. In addition, the alveolar septum contained some smaller NP agglomerates.
The content of CeO2 in all extrapulmonary organ was very low according to the authors. Besides the lung associated lymph nodes, CeO2 burden was highest in liver (12 +/- 3 µg/organ) and relatively high amounts of Ce was found also in femur bones (~1.8 +/- 0.4 µg/g) and bone marrow (~0.350 +/- 0.1 µg/g) in animals of group 4 after 24 month of exposure in comparison to all other extrapulmonary organs, except LALNs. The burden of the skeleton (calculated from CeO2 concentration in femur tissue) in the same animal group was calculated to be 44.9 µg/skeleton. CeO2 burden in other organs were negligible. The following ranking was proposed by the authors lungs > lymph node > hard bone > liver > bone marrow > kidneys, spleen >>> heart > brain > olfactory bulb. According to the authors, under these assumptions, around 1.1% of the lung burden would be retained in the skeleton for the group 4. In comparison, 1.2% of the lung burden was found in all investigated extra-pulmonary organs excluding lymph nodes and skeleton.
According to the accumulation rates, the authors concluded that, with a low accumulation rate, the liver can be regarded as a depot, whereas kidneys, the skeleton and bone marrow seem to be dumps due to steadily increasing nanoparticle burden over time.
It should be noted that in all examined extrapulmonary organs and histopathological examinations of the rats of this study, there was no detection of any adverse effects and no indications of systemic toxicity were found in any of the examined organs (see Keller et al, 2015 and Schaudien et al, 2019, in IUCLID section 7.5.2.
- Endpoint:
- basic toxicokinetics in vivo
- Type of information:
- experimental study
- Adequacy of study:
- weight of evidence
- Study period:
- Not applicable
- Reliability:
- 2 (reliable with restrictions)
- Rationale for reliability incl. deficiencies:
- study well documented, meets generally accepted scientific principles, acceptable for assessment
- Remarks:
- ; however, the physico-chemical characterisation was performed on non-radioactive nano-CeO2 instead of the radioactive nanoparticles which were the material administered to animals.
- Reason / purpose for cross-reference:
- reference to same study
- Reason / purpose for cross-reference:
- reference to same study
- Reason / purpose for cross-reference:
- reference to other study
- Objective of study:
- bioaccessibility (or bioavailability)
- distribution
- excretion
- toxicokinetics
- other: clearance
- Qualifier:
- no guideline followed
- Principles of method if other than guideline:
- - Single oral (gavage) administration of a suspension of nanometric cerium dioxide (nano-CeO2) to rats; sacrifice of animals 5 min and 7 days post-dosing
- Bioaccessibility: Scanning electron microscopy (SEM), analytical ultracentrifugation (AUC), laser diffraction (LD), inductively coupled plasma-mass spectrometry (ICP-MS)
- Biodistribution, clearance and excretion: Radioactivity measurement using a gamma counter - GLP compliance:
- not specified
- Radiolabelling:
- yes
- Remarks:
- neutron-activated 141CeO2
- Species:
- rat
- Strain:
- Wistar
- Sex:
- male
- Details on test animals or test system and environmental conditions:
- TEST ANIMALS
- Source: Charles River Laboratories (USA)
- Age at study initiation: 8 weeks old
- Weight at study initiation, fasting period before study: No data available
- Housing: In standard microisolator cages
- Individual metabolism cages: No data available
- Diet: Ad libitum; commercial chow (PicoLab Rodent Diet 5053, USA)
- Water: Ad libitum; reverse-osmosis purified water
- Acclimation period: At least 7 days before the start of experiments
ENVIRONMENTAL CONDITIONS
- Temperature, humidity: Controlled conditions of temperature and humidity
- Air changes: No data available
- Photoperiod: Controlled conditions of light
IN-LIFE DATES: No data available - Route of administration:
- oral: gavage
- Vehicle:
- water
- Details on exposure:
- PREPARATION OF DOSING SOLUTIONS:
Particle suspensions at specified concentrations were prepared in sterile distilled water in conical polyethylene tubes. Critical sonication energy to achieve the smallest particle agglomerate size was used. The suspensions were sonicated at in a cup sonicator. The sample tubes were immersed in running cold water to minimize heating of the particles during sonication. The hydrodynamic diameter (HD), polydispersity index (PdI) and zeta potential (ζ) of each suspension were measured by dynamic light scattering (DLS). In addition, the radioactivity in multiple aliquots of each suspension or solution was measured in a Gamma Counter.
VEHICLE
- Concentration in vehicle: 5 mg/mL
- Amount of vehicle: 1 mL/kg
- Lot/batch no, purity: No data available
HOMOGENEITY AND STABILITY OF TEST MATERIAL:
No data available
ORAL ADMINISTRATION
Each rat was anaesthetized with isoflurane. The 141nano-CeO2 suspension was delivered into the stomach via the oesophagus. Each rat was then placed in a metabolic cage with food and water ad libitum for faecal and urine sample collection. Rats (5/gp) were humanely killed 5 min and 7 days post-gavage. - Duration and frequency of treatment / exposure:
- A single exposure (sacrifice 5 min and 7 days post-dosing)
- Dose / conc.:
- 0 mg/kg bw (total dose)
- Remarks:
- (control)
- Dose / conc.:
- 5 mg/kg bw (total dose)
- Remarks:
- basis: nominal nano-CeO2 conc.
- No. of animals per sex per dose / concentration:
- At least 5
- Control animals:
- yes, concurrent vehicle
- Details on study design:
- - Dose selection rationale, rationale for animal assignment: No data available
- Details on dosing and sampling:
- PHARMACOKINETIC STUDY (Absorption, distribution, excretion)
Analysis of rats at 5 minutes post-gavage was performed to obtain an accurate measure of the initial deposited dose. Since the authors anticipated that clearance from the gastrointestinal (GI) tract would be faster, the gavage experiment spanned only 7 days.
- Tissues and body fluids sampled: urine, faeces, blood, plasma, serum, lung, bone marrow, femoral bone, skin, brain, skeletal muscle, testicles, kidneys, spleen, heart, liver, stomach, small intestine, large intestine, caecum
- Time and frequency of sampling: Twenty four-hour samples of faeces and urine were collected at selected time points (0–24 hours, 2–3 days and 6–7 days post-gavage). The other tissues were sampled immediately and 7days post-administration.
At each time point, rats were anesthetized and as much blood as possible was collected from the abdominal aorta. Plasma and red blood cells were separated by centrifugation. Each sample weight was recorded. Radioactivity was measured in a Gamma Counter. Disintegrations per minute were calculated from the measured counts per minute (minus background) and the counter efficiency. Data were expressed as μCi/g and as a percentage of the administered dose retained in each organ. All radioactivity data were adjusted for physical decay over the entire observation period. The radioactivity in organs and tissues not measured
in their entirety was estimated from measured aliquots as a percentage of total body weight as follows: skeletal muscle, 40%; bone marrow, 3.2%; peripheral blood, 7%; skin, 19%; and bone, 6%.
OTHER
IN VITRO BIOACCESSIBILITY TESTS:
Nano-CeO2 was incubated under varying pH and chemical conditions to measure bioaccessibility, which is the fraction of cerium (Ce) that dissolves. The nanoparticles were incubated in different fluids at 37 °C: phosphate-buffered saline (PBS) for 28 days to simulate surface deposition in the lungs, synthetic phagolysosomal simulant fluid (PSF) for 28 days to simulate uptake and digestion in macrophages, 0.1N HCl for 1 day to simulate oral ingestion and stomach transit time, and fasted state simulant intestinal fluid (FaSSIF) for 7 days to simulate GI intraluminal conditions. All incubation times were chosen at or above the maximum realistic residence time of nano-CeO2 in specific body compartments, since the authors expected low dissolution in these simulant fluids. Nanoparticle agglomeration (size and charge), release of ions in supernatant by inductively coupled mass spectroscopy (ICP-MS), structural changes of particles by scanning and transmission electron microscopy (SEM/TEM) on sediment and nanoparticle durability by selected area electron diffraction (SAD) were all determined. - Statistics:
- All data were analysed using multivariate analysis of variance (MANOVA) followed by Bonferroni (Dunn) post hoc tests.
- Preliminary studies:
- No data available
- Details on absorption:
- Nano-CeO2 bioavailability from the gut was orders of magnitude less than that via the lungs (see data in ESR entitled "Nano-CeO2 - biodistribution in rats - i.t. - V2 2014Moli").
- Details on distribution in tissues:
- In both studies, nearly 100% of the dose was recovered in the stomach at 5 min after gavage. Very low levels of 141Ce from nanoparticles were measured in all organs examined at 7 days post-gavage: e.g., maximum of 0.003 ± 0.0004% in all organs combined (see in Table 1 below for the Molina et al, (2014 study) and 0,004 % in the Konduru et al (2015) study.
- Details on excretion:
- Nearly the entire 141Ce dose from 141nano-CeO2 was rapidly excreted in the faeces (ca. 75% of the administered dose at day 1 post-gavage, ca. 90% at day 3, and ca. 95-99% at day 7) and urine (< 0.005% of the administered dose) over 7 days in both studies.
- Metabolites identified:
- not measured
- Bioaccessibility (or Bioavailability) testing results:
- IN VITRO TESTS (Molina et al., 2014):
Nano-CeO2 was virtually insoluble under all conditions tested. The nanoparticles formed agglomerates in most physiological simulant fluids. The fine fraction (defined as particle or agglomerate with diameter below 1 μm, determined by AUC) decreased significantly in physiological media when compared to distilled water. Under all conditions, the primary particles remained recognizable in SEM scans, but in buffers with organic constituents (PSF, FaSSIF) the surface charge reversed to negative zeta-potentials, which indicated adsorption of buffer constituents to the nanoparticles. The nano-CeO2 showed structural changes in the acidic PSF medium. The SEM scan after the 28-day incubation in PSF showed micron-sized spheres and greater than 100 nm rhombohedron crystallites that were absent in the as-produced powder. SAD analysis showed that these structures retained the same cubic cerianite crystalline phase of the as-produced powder. - Conclusions:
- In both studies, the authors concluded that risk from ingested nano-CeO2 was likely very weak due to very low absorption and rapid elimination of CeO2 not absorbed from the gastrointestinal tract.
=> Interpretation of results: low bioaccumulation potential based on study results - Executive summary:
Molina RM et al. (2014) and Konduru et al. (2015) from the same group, investigated the bioavailability, tissue distribution, clearance and excretion of radioactive 141cerium (141Ce) after gavage of neutron-activated141 nanometric cerium dioxide (141nano-CeO2) in Wistar Han rats.
A commercial nano-CeO2 (NM-212) provided by Mercator GmbH (Molina et al. 2014) and a in house synthesized nanoCeO2 (Konduru et al. 2015) were used in this study. Nano-CeO2 powder was neutron activated; the process generated the radioisotope 141Ce. The physico-characterisation was performed on non-radioactive NM-212, thus supposing that radioactive nano-CeO2 had the same physico-chemical properties as its stable counterpart. Nano-CeO2 NM-212 displayed a primary particle size of 40 nm and the in-house synthesised 32.0 nm. When suspended in distilled water, NM-212 had a hydrodynamic diameter of at least 207 nm. Thus, the suspended nanoparticles agglomerated in aqueous suspension. The specific surface area were 27 and 28 m²/g for the NM-212 and in house synthesised nanoCeO2, respectively . The surface charge of NM-212 was comprised between +37 and +42 mV, depending on the physico-chemistry technique employed; but the suspended nano-CeO2 was always cationic in water and the suspension displayed a pH of 7.85. These roughly globular particles were crystalline (> 99% pure cerianite). The surface chemistry of nano-CeO2 NM-212 was composed of 79.9%, 17.7% O and 2.4% Ce, but total content of 0.7% organic contaminations, identified as ester + alkyl groups, was found on the particle surface as a very thin and homogeneous layer around the pure inorganic particles. Moreover, the oxidation degree of NM-212 surface was mainly as Ce(IV) but 14% were found as Ce(III). In addition, the NM-212 particles were virtually insoluble under all conditions tested: for 24 h in DMEM with 10% foetal calf serum (FCS), for 28 days in phosphate buffered saline (PBS, to simulate the surface of epithelium), 28 days in phagolysosomal simulant fluid (PSF, to simulate the phagolysosomes of macrophages), 1 day in 0.1N HCl (to simulate the stomach environment following oral intake), or 7 days in a simulated intestinal fluid (fasted state, FaSSIF). Nano-CeO2 was also found to be photocatalytically active.
Male rats were exposed once to 0 (control) or 5 mg/kg of nano-CeO2 by oral (gavage) route in both studies. Control rats were treated with sterile distilled water, being nano-CeO2 vehicle. All treated rats were sacrificed 5 min and 7 days post-administration. Then, the following tissue samples were collected: blood, plasma, serum, lung, bone marrow, femoral bone, skin, brain, skeletal muscle, testicles, kidneys, spleen, heart, liver, stomach, small intestine, large intestine, and caecum. Moreover, 24-h samples of faeces and urine were collected at selected time points (0–24 hours, 2–3 days and 6–7 days post-gavage). The cerium (Ce) content in the samples was determined by measuring the radioactivity (141Ce) using a gamma counter.
In both studies, five minutes after gavage, nearly 100% of the 141nano-CeO2 dose (5 mg/kg) was recovered in the stomach of exposed rats. Very low levels of 141Ce from nanoparticles were measured in all organs examined at 7 days (e.g., maximum of 0.003 to 0,004 % in all organs combined). Moreover, nearly the entire 141Ce dose from 141nano-CeO2 was rapidly excreted in the faeces (ca. 75% of the administered dose at day 1 post-gavage, ca. 90% at day 3, and ca. 95 to 99% at day 7) and urine (< 0.005% of the administered dose) over 7 days.
The authors concluded that ingested nano-CeO2 posed almost no risk of absorption since ingested nano-CeO2 was almost entirely excreted in the faeces. Thus, the risk of extrapulmonary cerium tissue accumulation and potential toxicity could be considered as very low and probably insignificant when nanoparticulate CeO2 is ingested. In conclusion, the tested nano-CeO2 displayed the classical behaviour of poorly soluble particles: low bioaccumulation and high faecal excretion.
- Endpoint:
- basic toxicokinetics in vivo
- Type of information:
- experimental study
- Adequacy of study:
- weight of evidence
- Study period:
- Not applicable
- Reliability:
- 2 (reliable with restrictions)
- Rationale for reliability incl. deficiencies:
- study well documented, meets generally accepted scientific principles, acceptable for assessment
- Remarks:
- ; nevertheless the test was not conducted under GLP conditions. Moreover, there were some discrepancies regarding the number of test animals described in the "Materials & Methods" section and the figure legends.
- Reason / purpose for cross-reference:
- reference to other study
- Reason / purpose for cross-reference:
- reference to other study
- Reason / purpose for cross-reference:
- reference to other study
- Reason / purpose for cross-reference:
- reference to other study
- Reason / purpose for cross-reference:
- reference to other study
- Objective of study:
- other: blood, clot and serum distribution; rate of clearance from blood; comparison between sizes and shapes of nano-CeO2, and to metal form
- Qualifier:
- no guideline followed
- Principles of method if other than guideline:
- - Unique intravenous (i.v.) injection of either a suspension of nanometric cerium dioxide (nano-CeO2) with various physico-chemical properties, or Ce ion (as chloride); sampling at 0.167, 0.5, 0.75, 1, 2, 4, 20, 168 (1 week) or 720 hours (1 month) post-infusion and sacrifice of animals 168 or 720 hours after exposure
- Blood distribution/clearance: inductively coupled plasma mass spectrometry (ICP-MS) and pharmacokinetic modelling - GLP compliance:
- not specified
- Remarks:
- The GLP status was not specified in the article.
- Radiolabelling:
- no
- Species:
- rat
- Strain:
- Sprague-Dawley
- Sex:
- male
- Details on test animals or test system and environmental conditions:
- TEST ANIMALS
- Source: University of Kentucky (USA)
- Age at study initiation: No data available
- Weight at study initiation: 325 ± 30 g
- Fasting period before study: No data available
- Housing: Individually
- Individual metabolism cages, diet, water, acclimation period: No data available
ENVIRONMENTAL CONDITIONS
No data available
IN-LIFE DATES: No data available - Route of administration:
- intravenous
- Vehicle:
- water
- Details on exposure:
- Rats were prepared with two cannulas, surgically inserted into femoral veins under ketamine/xylazine anaesthesia, which terminated in the vena cava, and were connected to infusion pumps via a flow-through swivel. This enabled conduct of the study in the awake, mobile rat. All samples were sonicated to ensure dispersion prior to administration. The day after cannula implantation the non-anesthetized rat was infused via the longer cannula with cerium ion (Ce, as chloride), nano-CeO2 as a ~5 % nano-CeO2 suspension in water, or water.
Each of the materials was infused over 1 h, except for Ce ion that was infused over 2 h.
Control rats received water adjusted to the pH of the paired nano-CeO2 or Ce ion.
To compensate for the i.v. administration of a considerable volume of grossly hypotonic infusion, concurrent i.v. infusion of an equal volume and rate of 1.8% sodium chloride in water was delivered into the second, shorter, cannula. Each fluid was delivered at approximately 0.6 mL/h. - Duration and frequency of treatment / exposure:
- A single exposure (sampling performed 0.167, 0.5, 0.75, 1, 2, 4, 20, 168 (1 week) or 720 hours (1 month) after i.v. infusion; sacrifice 168 or 720 hours after i.v. infusion)
- Dose / conc.:
- 78 mg/kg bw (total dose)
- Remarks:
- for Ce55 in the preliminary study
basis: nominal nano-CeO2 dose - Dose / conc.:
- 100 mg/kg bw (total dose)
- Remarks:
- for Ce30 and Ce55 in the preliminary study
basis: nominal nano-CeO2 dose - Dose / conc.:
- 175 mg/kg bw (total dose)
- Remarks:
- for Ce5 in the preliminary study
basis: nominal nano-CeO2 dose - Dose / conc.:
- 250 mg/kg bw (total dose)
- Remarks:
- for Ce5, Ce15, and Ce55 in the preliminary study
basis: nominal nano-CeO2 dose - Remarks:
- CeM was not included in the preliminary study.
- Dose / conc.:
- 0 mg/kg bw (total dose)
- Remarks:
- (control)
in the main study - Dose / conc.:
- 100 mg/kg bw (total dose)
- Remarks:
- for Ce5, Ce15 and Ce30 in the main study
basis: nominal nano-CeO2 dose
This corresponded to an analytical dose of 85 mg/kg for Ce5 and Ce30, and 70 mg/kg for Ce15. - Dose / conc.:
- 50 mg/kg bw (total dose)
- Remarks:
- for Ce55 in the main study
basis: nominal nano-CeO2 dose
This corresponded to an analytical dose of 50 mg/kg. - No. of animals per sex per dose / concentration:
- - Ce ion (for measurements of cerium concentration in whole blood):
* n = 3 for all time points
- Ce5 (for measurements of cerium concentration in whole blood):
* n = 9 at 0.167 h post-infusion
* n = 10 at 0.5 h post-infusion
* n = 5 at 0.75 h post-infusion
* n = 21 at 1 h post-infusion
* n = 10 at 2 h post-infusion
* n = 5 at 4 h post-infusion
* n = 12 at 20 h post-infusion
* n = 5 at 168 h post-infusion
* n = 7 at 720 h post-infusion
- Ce15 (for measurements of cerium concentration in whole blood):
* n = 10 at 0.167 and 0.5 h post-infusion
* n = 5 at 0.75 h post-infusion
* n = 10 at 1, 2, and 4 h post-infusion
* n = 5 at 20, 168, and 720 h post-infusion
- Ce30 (for measurements of cerium concentration in whole blood):
* n = 6 at 0.167, 0.5, and 0.75 h post-infusion
* n = 10 at 1 h post-infusion
* n = 6 at 2 and 4 h post-infusion
* n = 8 at 20 h post-infusion
* n = 3 at 168 h post-infusion
* n = 11 at 720 h post-infusion
- Ce55 (for measurements of cerium concentration in whole blood):
* n = 5 at 0.167, 0.5, and 0.75 h post-infusion
* n = 10 at 1 h post-infusion
* n = 5 at 2 and 4 h post-infusion
* n = 7 at 20 h post-infusion
* n = 5 at 168 and 720 h post-infusion
- CeM (for measurements of cerium concentration in whole blood):
* n = 5 for all time points - Control animals:
- no
- Details on study design:
- A pilot study was conducted with the cerium ion and each of the nano-CeO2 to determine the tolerability following i.v. infusion. A 100 mg cerium ion/kg infusion was lethal. Rats were infused approximately 250 or 175 mg/kg of Ce5; three of eight died. A dose of approximately 250 mg of Ce15/kg was tolerated. Infusion of 100 mg/kg of the Ce30 resulted in some evidence of mild distress (tachypnea, skittish behaviour and not resting well). Dyspnoea and lethargy were seen in rats given 78, 100, or 250 mg of Ce55/kg. Therefore, the target doses were 100 mg/kg for the Ce5, Ce15, Ce30 and 50 mg/kg for the Ce55 and the cerium ion.
- Details on dosing and sampling:
- PHARMACOKINETIC STUDY (Absorption, distribution, excretion)
Blood was sampled at 0.167, 0.5, 0.75, 1, 2, 4, 20, 168, or 720 h post-injection. After infusion, blood (0.6 mL) was withdrawn at the different time points from the cannula that had not delivered Ce. The blood was immediately separated volumetrically into two aliquots to enable determination of Ce in whole blood in one and in the serum and clot fractions of the other.
To determine the distribution of Ce in the serum and clot fractions samples (0.3 mL) of whole blood was allowed to clot at room temperature, the clot given time to contract, and serum withdrawn into a digestion vessel. The clot was withdrawn and placed in another digestion vessel and the remaining serum added to the serum sample. This enabled determination of the mass amount of Ce in the two major compartments of blood; serum and, from the clot fraction, association with the formed elements (red cells, white cells and platelets).
To determine Ce in whole blood the sample was transferred into a polytetrafluoroethylene digestion vessel (CEM) to which trace-metal grade nitric acid and 30% H2O2 were added, digested in a microwave at 180°C for 10 min to convert all Ce to dissolved Ce4+, diluted in water, and subsequently further diluted dependent on the Ce concentration. Ce concentration was determined in whole blood, serum and clot by ICP-MS. A Method Detection Limit (MDL) of Ce for blood and serum samples of 0.018 mg/L was determined (see Yokel RA at al., 2009). No samples in this study had Ce concentrations below this MDL. Seven samples, containing whole blood, serum or clot, were analysed in duplicate and were spiked with 8.3 ng Ce/mL. Results of duplicate analysis showed a range of 0 to 4% between the two determinations. Recovery of the Ce spike ranged from 93 to 114%, averaging 105%.
OTHER (see below in “any information on materials and methods incl. tables)
- PHARMACOKINETIC MODELLING
- RATIO OF Ce CONCENTRATION IN WHOLE BLOOD FOLLOWING INFUSION OF NANO-CeO2 VERSUS Ce ION
- Ce PARTITIONING BETWEEN SERUM AND CLOT - Statistics:
- Blood, serum and clot Ce concentrations were normalized to an infusion dose of 100 mg CeO2/kg for all rats, based on determination of the actual Ce content of the infusions by ICP-MS analysis, and because a few materials were given at doses other than 100 mg/kg. This enabled direct comparison of results from the Ce ion, nano-CeO2 sizes and shapes.
Outliers identified by the Grubb's test were not used in the data analysis. Four percentages of the 600 whole blood, serum and clot samples were outliers by this test. - Preliminary studies:
- As detailed by Yokel RA et al. (2013), 3 of 8 rats that received Ce5 died. There was evidence of mild distress in rats that received Ce30 (tachypnea, skittish, not resting well) and significant toxicity in rats that received Ce55 (dyspnoea, lethargic).
Therefore, the target doses were 100 mg/kg (ca. 0.58 mM) for Ce5, Ce15, and Ce30 and 50 mg/kg for Ce55. This large CeO2 exposure was used to enhance the ability to detect Ce in the blood and organs up to the 30 days after the single nano-CeO2 infusion. The authors found that the % of Ce5 dose in the liver was comparable after a single i.v. administration of 11 mg CeO2/kg, 5 administrations of this dose given over consecutive days, and a single administration of 56 mg CeO2/kg (unpublished results).
Given the persistence of nano-CeO2 in the mammal model, it was anticipated that small frequent doses will accumulate so that a single large dose, as used in this study, models the result of this accumulation (e.g., multiple smaller doses). - Details on absorption:
- Following a single i.v. administration, no animal died prior to their planned termination. Up to the times studied in this study, there was generally a decrease of blood cerium (Ce). Ce15, Ce30, Ce55, and CeM were rapidly cleared from blood since ≤ 2% of nano-CeO2 was found in blood 0.167 h after infusion. However, the clearance of Ce ion and Ce5 was less effective. Indeed, 14% of Ce ion and 32% of Ce5 remained in whole blood 0.167 h after exposure. This was calculated from the measured blood Ce concentration compared to the Ce dose, based on the i.v. infusion of 100 mg CeO2/kg into the rat’s vascular system (ca. 7% of its body volume), which would introduce approximately 1163 mg Ce/L blood. The authors suggested that the greater extent of citrate coating on 5-nm CeO2 (and therefore its greater hydrophilicity) may have contributed to its slower clearance. It has to be noted that the blood levels of Ce15 and Ce30 increased over the first 4 h after their infusion; according to Dan M et al., this suggested a redistribution of nano-CeO2 over time.
- Metabolites identified:
- not measured
- Bioaccessibility (or Bioavailability) testing results:
- No data available
- Conclusions:
- In conclusion, the kinetics and distribution of cerium ion did not predict those of the tested nano-CeO2. Clearance of nano-CeO2 from blood was size-dependent since 5-nm nano-CeO2, being resistant to agglomeration, was cleared much more slowly from blood than larger nano-CeO2 which were very rapidly cleared from circulating blood. The distribution of nano-CeO2 between serum and the blood clot was also size-dependent.
=> Interpretation of results: bioaccumulation potential cannot be judged based on study results - Executive summary:
Dan M et al. (2012) conducted an in vivo study to characterise distribution in, and clearance from blood of nanometric cerium dioxide (nano-CeO2), with various physico-chemical properties following a single intravenous (i.v.) injection to rats.
Five nano-CeO2 were used in this study. The nanomaterials were physico-chemically characterised:
Parameters
Results
Methods
Ce5
Ce15
Ce30
Ce55
CeM
Primary particle size
Expected value
Measured average particle size
5 nm
4.6 ± 0.1 nm {4.8 nm}
15 nm
12.0 ± 0.2 nm {13.5 nm}
30 nm
31.2 ± 0.5 nm {51 nm}
55 nm
55.0 ± 0.2 nm {59 nm}
-
Cubic = 30 nm and rod = 10 nm diameter and 2 µm length
-
TEM
Particle size distribution in water - Number basis [Volume basis]
7 nm [98%, 7 nm; 2%, 18 nm]
25 nm [57%, 25 nm; 43%, 145 nm]
41 nm [36%, 41 nm; 64%, 273 nm]
No data
No data
TEM, dynamic light scattering (DLS)
Stability
Slight agglomeration/aggregation tendency
Slight agglomeration/aggregation tendency
Slight agglomeration/aggregation tendency
No data
No data
-
Specific surface area [mean diameter estimated from surface area]
121 m²/g [6.5 nm]
71 m²/g [11 nm]
15 m²/g [52 nm]
No data
Not determined
BET method
Surface charge in water at pH ~7.3 (zeta potential)
-53 ± 7 mV
-57 ± 5 mV
-56 ± 8 mV
-32 ± 2 mV
No data
DLS
Shape
Polyhedral
Polyhedral
Cubic
Polyhedral
Cubic and rod
TEM
Crystallinity
Crystalline (face-centred cubic)
Crystalline (face-centred cubic)
Crystalline (face-centred cubic)
Crystalline (face-centred cubic)
Crystalline (face-centred cubic)
X-ray diffraction (XRD), TEM
Cerium content in nano-CeO2 suspension
Analytical dose was 85% of the intended 100 mg/kg nominal dose.
70% of the intended 100 mg/kg nominal dose
85% of the intended 100, 100 mg/kg nominal
103% of the intended 50 mg/kg nominal dose
No data
ICP-MS
Surface properties – Extent of surface citrate coating
ca. 40% surface coated with citrate
ca. 27% surface coated with citrate
ca. 18% surface coated with citrate
ca. 15% surface coated with citrate
No data
Thermogravimetric analysis (TGA)
Oxidation degree
Enrichment of Ce3+ at the particle rim (surface layer)
Enrichment of Ce3+ at the particle rim (surface layer)
Enrichment of Ce3+ at the particle rim (surface layer)
Enrichment of Ce3+ at the particle rim (surface layer)
Enrichment of Ce3+ at the particle rim (surface layer)
Electron energy loss spectrometry (EELS)
Free Ce content in suspensions
11.6 ± 0.3%
<< 1%
<< 1%
<< 1%
No data
ICP-MS
pH of suspensions
pH 8-9
pH 3.5
pH 3.9
pH 7
No data
pH metry
{d} mean diameter estimated from TEM measurements
Male Sprague-Dawley rats (3 to 21/group) were i.v. infused with the tested nano-CeO2 (as a 5% suspension in water) at the nominal doses of 0 (control), 50 (Ce55) or 100 mg/kg (Ce5, Ce15, Ce30).Control rats received water, which was nano-CeO2 vehicle. In addition, Ce ion was administered to rats at a dose level of 50 mg/kg through i.v. infusion. Blood samples were collected at 0.167, 0.5, 0.75, 1, 2, 4, 20, 168 (1 week), and 720 h (1 month) after exposure. Animals were terminated 168 or 720 h later. Blood, serum and clot distribution of cerium (Ce) was assessed by inductively coupled plasma-mass spectrometry (ICP-MS). Moreover, a pharmacokinetic analysis of Ce concentrations in blood was performed, notably to compare the data for nano-CeO2 to those of Ce ion.
Regarding blood distribution and clearance of nano-CeO2, Ce5 was cleared much more slowly than larger nano-CeO2. Nano-CeO2 larger than 5 nm were rapidly cleared from circulating blood. All nano-CeO2 seemed to display a prolonged retention throughout the rat. Traditional pharmacokinetic modelling showed best fit for 5-nm nano-CeO2 and Ce ion. After initially declining, whole blood Ce15 and Ce30 increased over 4 h after infusion; these results were not well-described by traditional pharmacokinetic modelling). The distribution of nano-CeO2 between serum and the blood clot was size-dependent. Ce ion, and Ce5 and Ce55 did not preferentially distribute into serum or clot, CeM was predominantly in the clot, and Ce15 and Ce30 migrated into the clot over 4 h, suggesting a change in surface properties of Ce15 and Ce30. The latter nanomaterial showed the greatest distribution in the clot fraction. The authors suggested that reticulo-endothelial organs may not readily recognize Ce5. Increased cerium distribution into the clot over time may be due to opsonisation.
In conclusion, traditional pharmacokinetic analysis was not very informative and nano-CeO2 pharmacokinetics were quite different from that of Ce ion.
- Endpoint:
- basic toxicokinetics in vivo
- Type of information:
- experimental study
- Adequacy of study:
- weight of evidence
- Study period:
- Not applicable
- Reliability:
- 2 (reliable with restrictions)
- Rationale for reliability incl. deficiencies:
- study well documented, meets generally accepted scientific principles, acceptable for assessment
- Remarks:
- but test not conducted under GLP conditions.
- Reason / purpose for cross-reference:
- reference to other study
- Objective of study:
- other: brain capillary cell association and brain entry rate of nano-CeO2 in rat
- Qualifier:
- no guideline followed
- Principles of method if other than guideline:
- - In situ brain perfusion of a suspension of nanometric cerium dioxide (nano-CeO2) suspension in rats; sacrifice of animals at the end of perfusion
- Nano-CeO2 concentration, localization, and chemical speciation in the brain:
* inductively coupled plasma mass spectrometry (ICP-MS)
* light and electron microscopy (LM and EM)
* capillary depletion method
- Blood-brain barrier (BBB) integrity: brain perfusion of BBB vascular space markers and quantification of signal using fluorescence or ICP-MS - GLP compliance:
- not specified
- Remarks:
- The GLP status was not specified in the study.
- Radiolabelling:
- no
- Species:
- rat
- Strain:
- Sprague-Dawley
- Sex:
- male
- Details on test animals or test system and environmental conditions:
- TEST ANIMALS
- Source, age at study initiation: No data available
- Weight at study initiation: 330 ± 35 g
- Fasting period before study: No data available
- Housing: Individually
- Individual metabolism cages, diet, water, acclimation period: No data available
ENVIRONMENTAL CONDITIONS
No data available
IN-LIFE DATES: No data available - Route of administration:
- other: brain perfusion
- Vehicle:
- other: saline solution (see below)
- Details on exposure:
- Free cerium in nano-CeO2 suspension was removed by ultrafiltration, using 3 kDa molecular weight cut-off regenerated cellulose centrifugal filtration devices. Then, sodium citrate solution (0.17 M) was added to the filtration device, which was bath sonicated for 15 min to re-disperse the tested nano-CeO2.
The rat was anaesthetized using ketamine/xylazine (75 and 5 mg/kg), and its left carotid was exposed. PE60 tubing containing heparin (100 U/mL, in 0.9% NaCl) was inserted into the common carotid. The perfusate contained the required concentrations of nano-CeO2 in a solution of Na+ (153 mM), K+ (4.2 mM), Ca2+ (1.5 mM), Mg2+ (0.9 mM), Cl- (162 mM), and glucose (9 mM). The perfusate flow rate was of 20 mL/minutes. The rat was decapitated to end the perfusion, and the brain was harvested and cleaned of meninges and surface vessels.
* in situ brain perfusion of 100 µg/mL at 15 or 20 mL/min for 120 s
* in situ brain perfusion of 30, 100, and 500 µg CeO2/mL at 20 mL/min for 120 s
* in situ brain perfusion of 30 µg CeO2/mL at 20 mL/min for 20, 60, or 120 s - Duration and frequency of treatment / exposure:
- A single exposure (sacrifice at the end of perfusion)
- Dose / conc.:
- 0 other: µg/mL
- Remarks:
- (control)
- Dose / conc.:
- 30 other: µg/mL
- Remarks:
- basis: nominal nano-CeO2 concentration
- Dose / conc.:
- 100 other: µg/mL
- Remarks:
- basis: nominal nano-CeO2 concentration
- Dose / conc.:
- 500 other: µg/mL
- Remarks:
- basis: nominal nano-CeO2 concentration
- No. of animals per sex per dose / concentration:
- - Flow rate dependency experiments:
* control at 15 mL/min => n = 4
* control at 20 mL/min => n = 5
* 100 µg/mL at 20 mL/min => n = 10
- Concentration dependency experiments:
* 30 µg/mL at 20 mL/min for 120 s => n = 4
* 100 µg/mL at 20 mL/min for 120 s => n = 6
* 500 µg/mL at 20 mL/min for 120 s => n = 5
- Perfusion duration dependency experiments:
* 30 µg/mL at 20 mL/min for 20, 60, and 120 s => n = 4 (control: n = 3) - Control animals:
- yes, concurrent vehicle
- Details on dosing and sampling:
- PHARMACOKINETIC STUDY (Absorption, distribution, excretion)
- ORGAN WEIGHT/ DISTRIBUTION:
Only brain tissue (ca. 20 mg from each brain region, except the choroid plexus, which averaged 0.9 ± 0.4 mg) was collected from the frontal cortex, parietal cortex, occipital cortex, thalamus/hypothalamus, midbrain/colliculus, striatum, cerebellum, hippocampus, and choroid plexus, from the left hemisphere, for measurement of nano-CeO2 (as Ce) by ICP-MS.
- NANO-CeO2 ACCUMULATION IN THE BRAIN:
The capillary depletion method was used to separate brain parenchyma from capillary tissue. After nano-CeO2 perfusion, a 20-sec washout was conducted. The forebrain from the perfused hemisphere was isolated from 10 rats (2 control and 8 treated) and the lateral ventricle choroid in the perfused hemisphere was removed. After homogenization and centrifugation, the supernatant (brain-rich fraction) and pellet (capillary-rich fraction) were carefully separated for measurement of nano-CeO2 (as Ce) by ICP-MS. The method detection limit (MDL) of Ce in tissue was 0.013 mg Ce/kg.
Moreover, 3 rats were prepared for light microscopy (LM) and TEM examination, after perfusion with 100 µg CeO2/mL, 20 mL/min for 120 s. The rats were then decapitated at the end of the 20-second in situ washout perfusion (with CeO2-free perfusate) for brain removal. After bisection, each hemisphere was fixed. Tissue samples from the pituitary gland, hippocampus, and choroid plexus were identified and stored. Samples were cut (3 mm3) before being dehydrated and embedded. Then, the blocks were sectioned at a thickness of 1 µm and toluidine-blue stained for LM screening, and selected blocks were sectioned at a thickness of 80 nm for TEM at 80 keV.
OTHER
- BRAIN-BLOOD BARRIER (BBB) INTEGRITY:
To evaluate the BBB integrity, gadolinium-diethylenetriamine pentaacetic acid (Gd-DTPA) (1 mM) was added to the perfusate. Gd-DTPA is a marker for vascular space which does not cross the intact BBB, due to its charge and high molecular weight. A significant increase in Gd-DTPA in brain samples indicates BBB damage. Following at least 1-h incubation at 37°C, the perfusate was bubbled for 2 min with 95/5 air/CO2 and adjusted to pH 7.4 prior to its use. Gadolinium concentration was analysed by ICP-MS. The MDL of Gd in tissue was 0.0004 mg Gd/kg. - Statistics:
- The distribution volume, or uptake space (Q), derived from the in situ brain perfusion results, was the amount of brain tissue into which the substrate distributed during a given perfusion duration: QCeO2 total (mL/g) = nano-CeO2 in tissue (μg/g)/CeO2 per volume of perfusate (μg/mL). The mass amount of nano-CeO2 per gram of brain (μg/g) was also calculated, in order to compare the results of perfusion of the three nano-CeO2 concentrations. Gd-DTPA uptake space (vascular plus extracellular space) was used to correct nano-CeO2 brain uptake space results:
QGd-DTPA = Gd-DTPA in tissue (μg/g)/ Gd-DTPA per volume of perfusate (μg/mL). Corrected brain Qnano-CeO2 = QCeO2 total – QGd-DTPA. The corrected mass amount of nano-CeO2 per gram of brain (μg/g) = (QCeO2 total – QGd-DTPA) (mL/g) x CeO2 per volume of perfusate (μg/mL).
Grubbs’ test was used to determine outliers. Two-way ANOVA, followed by Bonferroni multiple comparisons, was used to test for significant differences among the three concentration groups and nine different regions.
The percentage of the capillary surface area that would be covered by nano-CeO2 was calculated as:
(number of nano-CeO2 in the perfused hemisphere x cross-sectional area of a CeO2 particle) x 100%/rat brain capillary endothelial cell surface area. This was based on the CeO2 average diameter, the CeO2 density = 7.132 g/cm3, and the rat’s capillary surface area = 140 cm²/g brain. All results were reported as mean ± standard deviation (SD). - Preliminary studies:
- No data available
- Details on absorption:
- No data available
- Details on distribution in tissues:
- - NANO-CeO2 ACCUMULATION IN THE BRAIN AND BBB INTEGRITY:
* Flow rate dependency experiments
Vascular space of the brain was measured using Gd-DTPA. Comparison of uptake space (Qnano-CeO2) using 15 and 20 mL/min flow rates did not show a significant flow rate effect on brain CeO2 uptake in any of the eight regions (data not shown). There was no significant difference in the vascular space with these two flow rates or between the control and treated groups. These data suggested that nano-CeO2 did not influence BBB integrity. However, the choroid plexus showed a trend towards increasing vascular space compared to the other eight brain regions. Therefore, a 20-mL/min flow rate was used for the rest of the study.
* Concentration dependency experiments
To investigate the 5-nm CeO2 brain entry rate, its uptake space (Qnano-CeO2) was determined for each brain region and for each of the three CeO2 perfusate concentrations. The uptake space significantly decreased with the increase in nano-CeO2 concentration in the perfusate. In the 30 µg/mL group, there were no significant differences among the nine regions. However, in the 100 and 500 µg/mL groups, choroid plexus showed a trend towards increasing uptake space. When CeO2 uptake into the brain was expressed as mass amount (µg/g), no significant differences were found among the three treatment concentrations. The CeO2 mass amount in the choroid plexus was significantly higher than in the eight brain regions, and it was significantly higher in the 100 and 500 µg/mL groups than in the 30 µg/mL one. The average CeO2 mass amount per brain hemisphere (0.85 g, excluding the choroid plexus) was 4.2 ± 0.7 µg.
* Perfusion duration dependency experiments
To verify whether the uptake space of nano-CeO2 rapidly saturated, CeO2 uptake space was determined for 30 µg CeO2/mL for 20-, 60-, and 120-second perfusion durations. There was a trend towards increasing uptake space of multiple brain regions from 20 to 60 seconds, but no differences between 60 and 120 seconds, suggesting that the uptake space of this nano-CeO2 saturated between 20 and 60 seconds. The increased uptake space of the 5-nm CeO2 particles suggested that nano-CeO2 either associated with capillary endothelial cells or entered the brain.
* Cerium concentration in the brain
Cerebral capillaries were isolated from brain parenchyma to investigate the distribution of nano-CeO2 between these two fractions. Less than 0.4% of the perfused nano-CeO2 dose was associated with cerebral capillaries and brain parenchyma. The separation revealed that a great majority of the nano-CeO2 (99.4 ± 1.1% of the total nano-CeO2 in the capillary-rich fraction plus brain-rich fraction) was associated with the capillary endothelial cells. Less than 0.003% of the nano-CeO2 dose was associated with the brain-tissue-rich fraction. These results suggested that most of the 4.2 ± 0.7 µg nano-CeO2 found in the perfused brain hemisphere were associated with the capillary endothelial cells, and very little nano-CeO2 passed through the endothelial cells into brain extracellular fluid or brain cells.
* Nano-CeO2 localization in the brain
LM examination of the choroid plexus revealed the presence of a cuboidal epithelial layer surrounding a core of capillaries, with some surrounding loose connective tissue. There appeared to be no physical injury from exposure to the high perfusate flow rate. The pyramidal cells appeared unaffected by in situ perfusion. In the posterior pituitary, capillaries were not affected by the high perfusate flow rate.
At the ultrastructural level (TEM), the endothelial lining of the choroid plexus appeared intact. Consistent with the high CeO2 concentration in the choroid plexus, electron-dense nano-CeO2 agglomerations were observed on the endothelial lining. Meanwhile, electron-dense non-agglomerated nano-CeO2 adhered to the endothelial lining in the hippocampal vessels and the pituitary gland vessels that lacked a BBB. Nano-CeO2 associated with the surface of brain capillary endothelial cells. No CeO2 particle was observed in any brain parenchyma. The ultrastructural data lend support to the calculation that 2.4 ± 0.4% of the luminal surface area of the capillary endothelial cells would be occupied by the 4.2 ± 0.7 µg nano-CeO2 per hemisphere measured in the uptake study.
The results demonstrated that the tested nano-CeO2 did not influence BBB integrity. - Details on excretion:
- No data available
- Metabolites identified:
- not measured
- Bioaccessibility (or Bioavailability) testing results:
- No data available
- Conclusions:
- When administered by in situ perfusion into brain, nano-CeO2 predominantly localized on the surface of the brain capillary cells and very little nano-CeO2 passed through the endothelial cells into brain extracellular fluid or brain cells.
=> Interpretation of results: no bioaccumulation potential into brain - Executive summary:
Dan M et al. (2012) investigated the potential association of a suspension of 5-nanometre cerium dioxide (nano-CeO2) with brain capillary cells and/or the possible crossing of the blood-brain barrier (BBB) when administering the particles by in situ perfusion into rat brain.
A saline suspension of 4.35% crystalline nano-CeO2 (8.7 x 10E16 particles/mL) was used in this study. The in-house synthesised nano-CeO2 had a primary particle size of ca. 5 nm, a polyhedral shape, a crystalline structure, a specific surface area of 121 m²/g and a high Ce(III) valence. The particles showed no or few agglomeration/aggregation tendency in aqueous solutions at physiological pH, probably due to citrate coating which stabilised the suspended particles. The zeta potential of the nano-CeO2 was of -53 mV, at pH 7.35. Except traces of lanthanum, no contamination was detected in the nano-CeO2 suspension.
Nano-CeO2 uptake in the brain of Sprague-Dawley male rats was determined using the in situ brain perfusion technique at 15 and 20 mL/minute flow rates; 30, 100, and 500 µg/mL CeO2 perfused for 120 seconds at 20 mL/minute; and 30 µg/mL perfused for 20, 60, and 120 seconds at 20 mL/minute (n = 3 to 6 animals per group). Eight brain regions and a choroid plexus (ipsilateral to the perfusion) were collected. The capillary depletion method and light and electron microscopy were used to determine CeO2 capillary cell and brain parenchymal association and localization.
The vascular space was not significantly affected by brain perfusion flow rate or nano-CeO2, demonstrating that again nano-CeO2 did not influence blood-brain barrier integrity. Measurements performed using inductively coupled plasma mass spectrometry (ICP-MS) showed a trend towards increasing cerium concentration in the choroid plexus in the 100 and 500 µg/mL CeO2 perfusion groups. Whereas Ce uptake was not significantly modulated in the other eight brain regions. CeO2 uptake into the eight brain regions was similar after 120-second perfusion of 30, 100, and 500 µg CeO2/mL. However, a trend towards increasing CeO2 uptake space was found in the eight brain regions and choroid plexus after 60 versus 20 seconds, and this parameter was similar after 60 and 120 seconds. The capillary depletion method showed that less than 0.4% of the perfused nano-CeO2 dose was associated with cerebral capillaries and brain parenchyma. Moreover, 99.4 ± 1.1% of the nano-CeO2 found in the brain associated with the capillary fraction. Electron microscopy showed that nano-CeO2 located on the endothelial cell luminal surface.
In conclusion, when administered by in situ perfusion into brain, nano-CeO2 predominantly localized on the surface of the brain capillary cells and very little nano-CeO2 passed through the endothelial cells into brain extracellular fluid or brain cells.
- Endpoint:
- basic toxicokinetics, other
- Remarks:
- Toxicokinetic assessment
- Type of information:
- other: Based on published data and toxicological studies
- Adequacy of study:
- other information
- Reliability:
- 2 (reliable with restrictions)
- Rationale for reliability incl. deficiencies:
- data from handbook or collection of data
- Executive summary:
Several toxicokinetic experimental data on stable and radioactive cerium dioxide and other more soluble cerium compounds were considered all together to evaluate the toxicokinetic behaviour of cerium dioxide. These data, together with available information on the physicochemical characteristics and the toxicological information on cerium dioxide, were used to perform a qualitative assessment of the absorption, distribution/metabolism and elimination/excretion of bulk cerium dioxide.
Regarding the absorption of bulk cerium dioxide, observed absorption of soluble radioactive cerium salts (nitrate and chloride) after oral exposure of rodents was found to be < to 0.1 % of the administered dose. Therefore, the systemic availability after oral exposure of bulk cerium oxide, that israther insoluble in water as illustrated by its very low water solubility (< 0.123μg/L at 20°C and at pH between 6.01 and 6.41), and for which no Kow can be determined (inorganic substance),cannot be greater than the soluble species investigated in this assessment and thus, is considered as negligible.
After inhalation, around 80 to 90 % of the inhaled bulk cerium dioxide is rapidly cleared from the body (within 0 to 7 days) and the remaining fraction deposited into the lung will clear more slowly(several days/months). Cerium deposited in the alveolar region can be cleared to the gastrointestinal tract via mucociliary movement and thus eliminated via feces, or to the lymph nodes, or absorbed into the systemic circulation. The studies suggest a low systemic absorption. However, none of the studies reported in this assessment estimated the exact absorbed fraction of CeO2. However, this fraction can be estimated from the results to be<10 %as a worst case, if it is considered that almost all of the deposited lung fraction will be totally absorbed.
The absorption of bulk cerium dioxide via the dermal route is expected to be rather low, if not negligible (<< 0.1 %) based on data on soluble cerium compounds or nano forms of cerium dioxide.
Regarding the distribution of bulk cerium dioxide, from the results reported in this assessment, it can be concluded that, once absorbed, cerium compounds seem to be distributed mainly in liver, skeleton and to a lesser extent spleen and kidney whatever the route of exposure.
As an element, cerium is neither created nor destroyed within the body. The cerium compounds may be altered as a result of various chemical reactions within the body, particularly dissolution and precipitation (with e.g. phosphates) but data have not demonstrated a change in the oxidation state of the cerium cation.
From the studies reported in this assessment, it appear that cerium is rapidly eliminated from the body after oral exposure (> 99.9 %) and after inhalation exposure (initial short-term clearance of ~ 75 to 95 %), mostly via faeces and under an unmodified form,but small amounts can also be eliminated via urine after inhalation exposure
Referenceopen allclose all
- CeO2 BIODISTRIBUTION:
Table 2: Estimated percentage (%) of nano-CeO2 Ce5 and Ce30 dose in organs and blood
Ce5 |
|
Ce30 |
|
|
|
No of exposure |
One (11 mg/kg) |
Five (11 mg/kg daily) |
One (6 mg/kg) |
|
|
Sacrifice time point |
30 d post-expo |
30 d post-expo |
1 h post-expo |
30 d post-expo |
90 d post-expo |
Liver |
40* |
30 to 40* |
70* |
40 to 50 |
20 to 30*# |
Bone marrow |
20 |
10 to 20 (± 30) |
1 to 2 (± 1) |
5 to 6 (± 3) |
5 |
Spleen |
2 to 3* (± 1) |
3 |
4 (± 3) |
3 to 4 (± 1) |
3 to 4* (± 1) |
Skeletal system |
2 to 3 |
1 to 2 (± 1) |
0.4 to 0.5 (± 0.4) |
1 (± 0.8) |
1 to 2 (± 1) |
Lung |
0.2 to 0.3* |
2 (± 2) |
2 (± 2) |
0.4 to 0.5 (± 0.6) |
0.2 (± 0.1) |
Skeletal muscle |
0.4 to 0.5 (± 0.4) |
0.5 to 0.6 (± 0.5) |
0.3 to 0.4 (± 0.1) |
0.3 (± 0.2) |
0.3#& |
Lymph node |
nd |
nd |
0.02 to 0.03 (± 0.03) |
0.04 to 0.05 (± 0.03) |
0.1 to 0.2 (± 0.3) |
Fat |
2 (± 2) |
0.3 (± 0.3) |
nd |
nd |
nd |
Kidney |
1 (± 1) |
0.1 to 0.2 (± 0.05) |
0.01 to 0.02 (± 0.01) |
0.1 to 0.2 (± 0.1) |
0.01 to 0.02 (± 0.01) |
Heart |
0.5 to 0.6 (± 0.5) |
0.04 (± 0.06) |
0.01 (± 0.005) |
0.1 (± 0.15) |
0.002 to 0.003 (± 0.003) |
Blood |
0.02 (± 0.01) |
0.003 (± 0.004) |
0.1 to 0.2 (± 0.1) |
0.06 (± 0.06) |
0.007 (± 0.003) |
Thymus |
0.1 (± 0.1) |
0.01 (± 0.01) |
0.01 (± 0.02) |
0.001 (± 5 x 10E-4) |
0.007 (± 0.0035) |
Brain |
0.002 to 0.003 (± 0.001) |
0.001 (± 8 x 10E-4) |
0.006 to 0.007 (± 0.004) |
0.03 to 0.04 (± 0.04) |
0.004 (± 0.003) |
Adrenal |
nd |
nd |
0.002 to 0.003 (± 0.001) |
0.001 to 0.002 (± 0.001) |
0.001 to 0.002 (± 0.001) |
*: significantly different from controls (data not shown)
#: significantly different from data measured at 1 h post-infusion
&: significantly different from data measured at 30 d post-infusion
nd: not determined
Table 3: Estimated percentage (%) of nano-CeO2 CeR dose in organs and blood
CeR |
|
|
|
No of exposure |
One (50 mg/kg) |
One (20 mg/kg) |
One (50 mg/kg) |
Sacrifice time point |
1h post-expo |
30 d post-expo |
30 d post-expo |
Liver |
40 to 50* (± 20) |
20* (± 5) |
20* |
Bone marrow |
3* (± 1) |
10# (± 5) |
40 to 50*#& (± 5) |
Spleen |
2 to 3* (± 1) |
10 to 20*# (± 5) |
10 to 20*# |
Skeletal system |
0.06 (± 0.04) |
4 (± 1) |
3 (± 1) |
Lung |
3 (± 2) |
0.5 (± 0.2) |
0.8 (± 0.2) |
Skeletal muscle |
0.6 (± 0.4) |
0.6 to 0.7 (± 0.4) |
0.7 (± 0.3) |
Fat |
nd |
0.1 to 0.2 (± 0.1) |
nd |
Lymph node |
nd |
nd |
0.08 (± 0.02) |
Blood |
0.4 to 0.5 (± 0.4) |
0.04 to 0.05 (± 0.1) |
0.003 to 0.004 (± 0.004) |
Thymus |
0.1 to 0.2 (± 0.1) |
0.007 (± 0.007) |
0.003 (± 0.002) |
Brain |
0.01 to 0.02 (± 0.01) |
0.003 (± 0.003) |
0.004 (± 0.004) |
*: significantly different from controls (data not shown)
#: significantly different from data measured at 1 h post-infusion
&: significantly different from data measured at 30 d post-infusion with a dose of 20 mg/kg
nd: not determined
Table
4: Results of relative deposition index (RDI) analysis. x = organs
showing both RDI > 1 and a partial chi-squared value for that organ
representing > 10% of the sum of the partial chi-squared values
|
Ce5 |
|
Ce30 |
|
|
CeR |
|
|
|
11 mg/kg (single dose) |
55 mg/kg (repeated dose) |
6 mg/kg |
|
|
50 mg/kg |
20 mg/kg |
50 mg/kg |
|
Day 30 |
Day 30 |
1 h |
Day 30 |
Day 90 |
1 h |
Day 30 |
Day 30 |
Liver |
X |
X |
X |
X |
X |
X |
|
|
Bone marrow |
X |
X |
|
|
|
|
|
X |
Spleen |
X |
|
X |
X |
X |
|
X |
X |
Table 1: Ce concentrations in blood (mg/L(a) and as a [% of nano-CeO2 dose]), brain, liver, and spleen (mg/kg wet weight and as a [% of nano-CeO2 dose]) of treated rats sacrificed at 1 and 20 h post-injection (2010)
CeO2 dose (mg/kg) |
Blood |
Brain |
Liver |
Spleen |
1-h termination |
|
|
|
|
0 (n = 7) 100 (n = 11) |
0.02 ± 0.03 270 ± 79 [27 ± 8] |
0.09 ± 0.10 2.6 ± 0.9 [0.020 ± 0.007] |
0.30 ± 0.54 424 ± 297 [22 ± 14] |
(b) 1765 ± 1915 [5 ± 6] |
20-h termination |
|
|
|
|
0 (n = 8) 100 (n = 12) |
(b) 12 ± 9 [1 ± 1] |
(b) 0.58 ± 0.15 [0.0046 ± 0.0011] |
(b) 1007 ± 264 [51 ± 11 ] |
(b) 2885 ± 882 [10 ± 3] |
(a): based on blood volume of the rat of 7% body weight and brain, liver, and spleen CeO2 concentrations x organ weights.
(b): all values were below the MDL.
The brain Ce concentration 1 h after infusion of this 5-nm CeO2 (2.6 mg/kg) was greater than the concentration measured 20 h after infusion (0.6 mg/kg). According to Hardas SS et al., it was likely that CeO2 in the bulk brain samples reflected CeO2 in the vascular bed (270 mg/kg at 1 h). The authors did not wash out the blood from the brain prior to decapitation. The product of Ce concentration in blood (270 mg/kg at 1 h) x vascular volume of the brain (2%) could account for the Ce in the bulk brain samples (2.6 mg/kg).
Table 2: Ce concentrations [Ce] (mg/kg wet weight and as a % of nano-CeO2 dose) in blood, brain, and liver of treated rats sacrificed at day 30 post-injection (2012)
Blood |
Brain |
Liver |
|
[Ce] % dose |
0.11 ± 0.16 0.01 ± 0.02 |
0.38 ± 0.52 0.008 ± 0.009 |
505 ± 238 44 ± 27 |
Table 2: Tissue concentration (µg/g wet tissue) and distribution (%) of CeO2 at 6 h, 1, 7 and 28 days post-instillation of radioactive nano-CeO2 (200 µg)
Tissue |
Ce content (µg/g) |
|
|
|
Ce distribution (%) |
|
|
|
6 h post-instil. |
1 d post-instil. |
7 d post-instil. |
28 d post-instil. |
6 h post-instil. |
1 d post-instil. |
7 d post-instil. |
28 d post-instil. |
|
Lung |
100 to 120 |
100 to 120 |
100 |
80 to 100 |
70 to 80 |
70 to 80 |
70 |
60 to 70 |
Stomach |
0.1 to 0.5 |
0.1 |
0.001 |
0.0005 |
0.1 |
0.01 to 0.1 |
0.0005 to 0.001 |
0.0005 to 0.001 |
Intestine |
0.1 |
0.005 to 0.01 |
0.001 |
0.0001 to 0.0005 |
< 10E-6 |
< 10E-6 |
< 10E-6 |
< 10E-6 |
Bone |
0.001 |
0.001 to 0.005 |
0.01 |
0.005 to 0.01 |
< 10E-6 |
< 10E-6 |
< 10E-6 |
< 10E-6 |
Spleen |
0.0005 |
0.001 |
0.001 |
0.05 |
5 x 10E-5 to 1 x 10E-4 |
0.0001 to 0.0005 |
0.0001 to 0.0005 |
0.01 |
Blood |
0.0005 |
0.0001 to 0.0005 |
0.0005 |
0.001 to 0.005 |
< 10E-6 |
< 10E-6 |
< 10E-6 |
< 10E-6 |
Liver |
0.0001 to 0.0005 |
0.001 |
0.005 |
0.01 to 0.05 |
0.0005 |
0.001 to 0.005 |
0.01 to 0.05 |
0.1 |
Heart |
0.0001 to 0.0005 |
0.0001 |
0.0001 to 0.0005 |
0.0001 to 0.0005 |
5 x 10E-5 to 1 x 10E-4 |
(1 to 5) x 10E-5 |
5 x 10E-5 to 1 x 10E-4 |
5 x 10E-5 to 1 x 10E-4 |
Kidney |
5 x 10E-5 to 1 x 10E-4 |
0.0001 to 0.0005 |
0.001 to 0.005 |
0.001 |
5 x 10E-5 |
0.0001 to 0.0005 |
0.001 to 0.005 |
0.001 to 0.005 |
Muscle |
5 x 10E-5 to 1 x 10E-4 |
0.0001 to 0.0005 |
5 x 10E-5 |
5 x 10E-5 to 1 x 10E-4 |
< 10E-6 |
< 10E-6 |
< 10E-6 |
< 10E-6 |
Brain |
(1 to 5) x 10E-5 |
0.0001 |
5 x 10E-5 to 1 x 10E-4 |
1 x 10E-5 |
(1 to 5) x 10E-5 |
5 x 10E-5 to 1 x 10E-4 |
5 x 10E-5 |
1 x10E-5 |
Testicle |
(1 to 5) x 10E-5 |
(1 to 5) x 10E-5 |
5 x 10E-5 to 1 x 10E-4 |
5 x 10E-5 to 1 x 10E-4 |
(1 to 5) x 10E-5 |
(1 to 5) x 10E-5 |
0.0001 |
0.0001 |
n = 6 per group
Chemical entity of Ce:
ToF-SIMS imaging mass spectrometry was used to answer the question in which chemical entity Ce was present in the femur sections of exposed rats ( group #4, 24 months of exposure). Via mass spectrometry, the authors could clearly identify and distinguish CeO+ ions and cerium phosphate ions from other ions like calcium phosphate. Large sized particule agglomerates from CeO2 and cerium phosphate were located in distinctive areas of the femur bone tissue sections. Whilst cerium phosphate locates predominantly in the trabeculae of the spongy bone section, the CeO2 NP agglomerates locate close to the bone marrow. The authors suggests that this indicates that the CeO2 NP might enter the bone through the bone marrow canals and afterward mineralize as cerium phosphate within areas where calcium phosphate is already present.
Tables:
Table 1:Lung burden - Predicted lung burden, clearance half-times and deposition fraction of exposed rats
Exposure time |
1day |
3 months |
12 months |
24 months |
|
Aerosol concentrations |
Measured lung burden (µg/lung) ± SD |
Predicted clearance t1/2 (days)* |
|||
Control |
- |
1.23 ± 0.63 |
1.56 ± 0.63 |
1.35 ± 036 |
- |
0.1 mg/m3 |
- |
19.92 ± 2.13 |
49.22 ± 11.46 |
78.02 ± 34.91 |
86 |
0.3 mg/m3 |
- |
48.47 ± 5.65 |
227.83 ± 27.71 |
348.4 ± 54.25 |
114 |
1.0 mg/m3 |
- |
258.14 ± 24.94 |
924.36 ± 99.96 |
1450.07 ± 275.03 |
164 |
3.0 mg/m3 |
- |
772.03 ± 126.45 |
2603.02 ± 337 |
4411.94 ± 1038.55 |
200 |
|
Predicted lung burden (µg/lung)* |
Deposition fraction (%)* |
|||
0.1 mg/m3 |
0.85 |
31.48 |
59.62 |
64.49 |
11.8 |
0.3 mg/m3 |
3.15 |
108.44 |
205.36 |
222.13 |
14.6 |
1.0 mg/m3 |
9.72 |
415.54 |
1170.92 |
1658.14 |
13.5 |
3.0 mg/m3 |
17.7 |
753.78 |
2124.03 |
3007.83 |
8.2 |
*Calculated with MPPD model.
Table2: Organ weights of the tissue, hard bone, bone marrow and feces collected during the study
Time points |
3 months |
12 months |
24 months |
||||||||||||
CeO2[mg/m³] exposure groups |
0 |
0.1 |
0.3 |
1 |
3 |
0 |
0.1 |
0.3 |
1 |
3 |
0 |
0.1 |
0.3 |
1 |
3 |
|
Organ weights and feces (g) +/- SD |
||||||||||||||
lung |
0,86 +/- 0.03 |
0.90 +/- 0.09 |
0.95 +/- 0.10 |
0.90 +/- 0.03 |
1.06 +/- 0.04 |
1.02 +/-0.09 |
1.05 +/- 0.09 |
1.00 +/- 0.01 |
1.09 +/- 0.02 |
1.17+/- 0.03 |
1.11 +/-0.04 |
1.12 +/- 0.05 |
1.12 +/-0.08 |
1.19 +/- 0.05 |
1.33 +/-0.17 |
olf.bulb |
0.08 +/- 0.01 |
0.08 +/- 0.01 |
0.09 +/- 0.01 |
0.09 +/- 0.01 |
0.08 +/- 0.01 |
0.07 +/- 0.02 |
0.07 +/- 0.01 |
0.06 +/- 0.01 |
0.06 +/- 0.01 |
0.07 +/- 0.01 |
0.08 +/- 0.00 |
0.62 +/- 0.35 |
0.83 +/- 0.09 |
0.87 +/- 0.04 |
0.42 +/- 0.43 |
Brain |
1.80 +/- 0.05 |
1.80 +/- 0.09 |
1.91 +/- 0.12 |
1.83 +/- 0.02 |
1.92 +/- 0.05 |
2.00 +/- 0.10 |
1.93 +/- 0.02 |
1.91 +/- 0.09 |
1.90 +/- 0.09 |
2.03 +/- 0.05 |
2.00 +/- 0.04 |
2.02 +/- 0.01 |
1.93 +/- 0.05 |
2.01 +/- 0.06 |
1.94 +/- 0.08 |
Lymph nodes (lung)
|
0.11 +/- 0.11 |
0.17 +/- 0.02 |
0.118 +/- 0.04 |
0.18 +/- 0.06 |
0.38 +/- 0.12 |
0.02 +/- 0.00 |
0.03 +/- 0.01 |
0.03 +/- 0.01 |
0.06 +/- 0.00 |
0.07 +/- 0.01 |
-* |
- |
- |
- |
- |
Tracheobronchial lymph nodes |
- |
- |
- |
- |
- |
- |
- |
- |
- |
- |
0.01 +/- 0.00 |
0.00 +/- 0.00 |
0.01 +/- 0.00 |
0.02 +/- 0.00 |
0.03 +/- 0.02 |
Mediastinal lymph nodes |
- |
- |
- |
- |
- |
- |
- |
- |
- |
- |
0.02 +/- 0.00 |
0.02 +/- 0.00 |
0.02 +/- 0.01 |
0.04 +/- 0.03 |
0.06 +/- 0.04 |
Mesenteric lymph node |
- |
- |
- |
- |
- |
0.27 +/- 0.11 |
0.28 +/- 0.11 |
0.25 +/- 0.07 |
0.19 +/- 0.01 |
0.22 +/- 0.03 |
0.29 +/- 0.08 |
0.17 +/- 0.05 |
0.24 +/- 0.13 |
0.18 +/- 0.01 |
0.17 +/- 0.08 |
Jejunum |
0.75 +/- 0.14 |
0.83 +/-0.09 |
1.05+/- 0.14 |
0.80+/- 0.04 |
0.85 +/- 0.05 |
1.20 +/- 0.14 |
0.95 +/-0.19 |
0.80+/- 0.21 |
1.00+/- 0.33 |
1.14+/- 0.46 |
0.50 +/- 0.27 |
0.46 +/- 0.05 |
0.57 +/- 0.20 |
0.47 +/- 0.12 |
0.49 +/- 0.26 |
Liver |
6.71 +/- 0.39 |
7.97 +/- 0.56 |
7.23 +/- 0.38 |
7.66+/- 0.58 |
8.36+/- 0.61 |
7.55 +/- 0.88 |
8.21 +/- 0.93 |
7.51+/- 0.92 |
8.30+/- 0.56 |
8.08+/- 0.70 |
9.10 +/- 0.21 |
10.21 +/- 1.23 |
9.20+/- 0.79 |
8.23+/- 0.61 |
8.61+/- 2.28 |
Kidneys |
1.33 +/- 0.09 |
1.41 +/- 0.09 |
1.49+/- 0.01 |
1.40 +/- 0.02 |
1.53 +/- 0.03 |
1.60 +/- 0.22 |
1.74 +/- 0.17 |
1.59+/- 0.11 |
1.61 +/- 0.13 |
1.74 +/- 0.04 |
2.20 +/- 0.01 |
2.02 +/- 0.13 |
2.09+/- 0.16 |
1.91 +/- 0.11 |
2.15 +/- 0.60 |
Spleen |
0.51 +/- 0.05 |
0.51 +/- 0.05 |
0.57 +/- 0.02 |
0.57 +/- 0.02 |
0.61 +/- 0.07 |
0.62 +/- 0.06 |
0.64 +/- 0.13 |
0.59 +/- 0.10 |
0.69 +/- 0.27 |
0.74 +/- 0.03 |
0.72 +/- 0.03 |
0.94 +/- 0.28 |
0.86 +/- 0.16 |
0.72 +/- 0.14 |
0.72 +/- 0.05 |
Heart |
0.65 +/- 0.03 |
0.67 +/- 0.05 |
0.76 +/- 0.05 |
0.71 +/- 0.04 |
0.76 +/- 0.03 |
0.79 +/- 0.07 |
0.84 +/- 0.04 |
0.78 +/- 0.04 |
0.79 +/- 0.08 |
0.78 +/- 0.03 |
1.14 +/- 0.00 |
0.95 +/- 0.08 |
1.02 +/- 0.09 |
0.94 +/- 0.09 |
0.97 +/- 0.10 |
Femura |
- |
- |
- |
- |
- |
- |
- |
- |
- |
- |
2.58 +/- 0.74 |
2.15 +/- 0.54 |
2.01 +/- 0.30 |
1.67 +/- 0.09 |
2.19 +/- 0.21 |
Bone marrow |
0.03 +/- 0.01 |
0.03 +/- 0.00 |
0.02 +/- 0.01 |
0.02 +/- 0.01 |
0.02 +/- 0.01 |
0.03 +/- 0.01 |
0.02 +/- 0.01 |
0.02 +/- 0.01 |
0.02 +/- 0.01 |
0.02 +/- 0.01 |
0.04 +/- 0.03 |
0.04 +/- 0.01 |
0.05 +/- 0.02 |
0.04 +/- 0.01 |
0.04 +/- 0.02 |
Blood |
-
|
-
|
-
|
-
|
-
|
-
|
-
|
-
|
-
|
-
|
1.87 +/- 0.01 |
1.90 +/- 0.08 |
1.85 +/- 0.09 |
1.94 +/- 0.05 |
1.94 +/- 0.07 |
Feces |
1.27 +/- 0.43 |
2.08 +/- 0.52 |
1.25 +/- 0.53 |
1.60 +/- 0.95 |
1.67 +/- 0.41 |
1.41 +/- 0.23 |
1.46 +/- 0.90 |
1.42 +/- 0.29 |
1.61 +/- 0.26 |
1.17 +/- 0.44 |
1.81 +/- 0.21 |
2.51 +/- 0.81 |
2.66 +/- 0.44 |
1.73 +/- 0.49 |
1.37 +/- 0.72 |
*: - = not measured
Table 3: CeO2organs burden
Months of exposure |
3 |
12 |
24 |
|||||||||
CeO2[mg/m³] exposure groups |
0.1 |
0.3 |
1 |
3 |
0.1 |
0.3 |
1 |
3 |
0.1 |
0.3 |
1 |
3 |
Organ burdens (µg or ng/organ or ng/g of tissue) |
||||||||||||
Lung-associated lymph node (LALN) µgCeO2/organ(mean +/- SD) |
0.01 +/- 0.01 |
0.11 +/- 0.01 |
1.33 +/- 0.85 |
30.39 +/- 0.00 |
0.61 +/- 0.50 |
18.55 +/- 5.93 |
165.68 +/- 45.11 |
646.67 +/- 144.43 |
0.33 +/- 0.17 |
12.59 +/- 7.65 |
572.45 +/- 140.71 |
2372.15 +/- 569.25 |
Mesenteric lymph node ngCeO2/organ(mean +/- SD) |
-* |
- |
- |
- |
2.17 +/- 0.10 |
4.03 +/- 1.52 |
16.26 +/- 7.29 |
48.36 +/- 10.44 |
2.06 +/- 0.59 |
7.88 +/- 4.63 |
39.26 +/- 23.57 |
72.93 +/- 19.96 |
Liver µgCeO2/organ (mean +/- SD) |
0.23 +/- 0.06 |
0.33 +/- 0.11 |
1.22 +/- 0.08 |
2.01 +/- 0.42 |
0.59 +/- 0.32 |
1.38 +/- 0.28 |
4.58 +/- 0.37 |
4.78 +/- 0.65 |
0.30 +/- 0.08 |
2.63 +/- 0.39 |
3.05 +/- 1.06 |
11.71 +/- 3.38 |
Speen ngCeO2/organ (mean +/- SD) |
0.93 +/- 0.35 |
1.75 +/- 0.72 |
4.37 +/- 2.25 |
48.51 +/- 4.11 |
5.86 +/- 5.44 |
20.93 +/- 9.33 |
155.31 +/- 34.26 |
169.57 +/- 14.25 |
19.77 +/- 4.18 |
75.89 +/- 42.59 |
281.59+/- 113.19 |
793.70 +/- 115.20 |
/Kidneys ngCeO/organ (mean +/- SD) |
9.19 +/- 3.80 |
26.30 +/- 2.12 |
16.62 +/- 3.86 |
39.11 +/- 7.77 |
31.53 +/- 3.03 |
50.87 +/- 1.76 |
118.80 +/- 21.17 |
217.69 +/- 41.34 |
53.93 +/- 7.64 |
211.85 +/- 75.73 |
317.84 +/- 86.10 |
854.46 +/- 386.64 |
Brain ngCeO2/organ(mean +/- SD) |
0.42 +/- 0.59 |
0.21 +/- 0.18 |
1.71 +/- 0.33 |
3.37 +/- 2.04 |
1.06 +/- 0.22 |
0.98 +/- 0.09 |
2.33 +/- 0.14 |
4.42 +/- 0.30 |
6.21 +/- 5.18 |
2.17 +/- 0.60 |
5.48 +/- 1.48 |
13.14 +/- 8.65 |
Heart ngCeO2/organ (mean +/- SD) |
0.63 +/- 0.29 |
0.72 +/- 0.09 |
1.60 +/- 0.36 |
4.23 +/- 1.32 |
1.75 +/- 0.17 |
3.64 +/- 0.60 |
7.37 +/- 0.81 |
11.87 +/- 3.50 |
8.68 +/- 5.05 |
11.08 +/- 2.77 |
30.53 +/- 17.90 |
58.22 +/- 16.38 |
Olfactory bulb ngCeO2/g tissue(mean +/- SD) |
0.16 +/- 0.16 |
0.18 +/- 0.02 |
0.40 +/- 0.06 |
2.52 +/- 1.78 |
0.22 +/- 0.05 |
0.42 +/- 0.02 |
1.00 +/- 0.39 |
1.79 +/- 0.32 |
0.42 +/- 0.11 |
1.29 +/- 0.16 |
2.11 +/- 0.75 |
8.98 +/- 5.07 |
Small intestine (jejunum) ngCeO2/g tissue(mean +/- SD) |
19.43 +/- 7.85 |
36.82 +/- 24.06 |
67.73 +/- 6.11 |
154.48+/- 60.17 |
9.15 +/- 1.08 |
22.30 +/- 0.69 |
28.84 +/- 9.09 |
174.07 +/- 183.51 |
3.87 +/- 1.76 |
11.89 +/- 6.26 |
39.06 +/- 14.12
|
167.15 +/- 123.62 |
Bone marrow ngCeO2/g tissue(mean +/- SD) |
11.19 +/- 4.04 |
5.95 +/- 2.66 |
8.01 +/- 5.68 |
31.28 +/- 11.51 |
29.36 +/- 14.73 |
30.28 +/- 10.61 |
99.08 +/- 50.79 |
213.40 +/- 25.77 |
26.46 +/- 14.91 |
70.46 +/- 14.59 |
228.44 +/- 105.58 |
349.30 +/- 108.22 |
µgCeO2/Skeleton** (mean +/- SD) |
- |
- |
- |
- |
- |
- |
- |
- |
3.83 +/- 1.15 |
9.77 +/- 2.09 |
24.22 +/- 4.97 |
44.89 +/- 8.57 |
µgCeO2/g of feces*** (mean +/- SD) |
~ 1 +/- 0.2 |
~ 1 +/- 0.0 |
~ 6 +/- 1 |
~ 28 +/- 0.0 |
~ 3.5 +/- 1 |
~ 5 +/- 1.5 |
~ 18 +/- 10 |
~ 46 +/- 14 |
~ 1 +/- 0.0 |
~ 3 +/- 0.5 |
~ 12.5 +/- 5 |
~ 16 +/- 6 |
*: - = not measured
**: CeO2bone (femur) burden (extrapolated based on the assumption that 6% BW of the rat equal the whole skeleton of the rat (Trevos 2006).
***: approximate concentrations deduced from the graph included in the article since exact values were not included under a table format.
Several toxicokinetic experimental data on stable and radioactive cerium dioxide and other more soluble cerium compounds were considered all together to evaluate the toxicokinetic behaviour of cerium dioxide. These data, together with available information on the physicochemical characteristics and the toxicological information on cerium dioxide, were used to perform a qualitative assessment of the absorption, distribution/metabolism and elimination/excretion of bulk cerium dioxide.
ABSORPTION
· Oral/gastro-intestinal (GI) absorption
Studies evaluating the absorption of cerium compounds following oral exposure in humans are not available.
Several animals’ studies, which used either radioactive cerium chloride or radioactive cerium nitrate (both being soluble forms of cerium), have been used to give information on the oral/gastrointestinal absorption of bulk cerium dioxide:
Inaba and Lengemann (1972) administered a single dose of [141Ce]-ceric nitrate of unreported concentration by intragastric dosing to 4 litters of Sprague-Dawley rats (n = 7–9), age 0, 7, 14, or 26 days, and to 2 litters of 1-day-old newborn rats. Then, whole-body radioactivity measurements or of the GI tract were taken immediately after dosing and periodically thereafter. In rats dosed at 26 days of age, only 0.04% of the administered radioactivity remained in the body by 3 days after dosing. In newborns, radioactivity diminished more slowly, dropping from 98% of administered dose on day 1 to 29% on day 16. The GI tract accounted for nearly 100% of whole-body retention on day 1 and 93% on day 16. After the onset of weaning in the suckling rats dosed as newborns, whole-body radioactivity fell to 3% of administered dose by day 24, of which only 17% was measured in the GI tract. Autoradiographs of the GI tract from the two litters dosed as 1-day-old newborns suggest rapid transit of radioactivity to the lower small intestine 1 day after exposure. Autoradiography of rats 5 days after dosing (6 days old) suggests that the intestinal radioactivity is restricted to the upper two-thirds of the epithelial villi, which the study authors associated with cerium concentration in the vacuoles.
Low absorption of soluble [144Ce]-ceric nitrate (unreported concentration) in adult female SD rats was also reported by Durbin et al. (1956): less than 0.1% of the intragastric-administered dose was absorbed from the GI tract 4 days after administration.
Similar results of absorption with cerium chloride were observed by Kostial et al. (1989b) and Shiraishi and Ichikawa (1972) who administered a single oral doses of an unreported concentration of [141Ce]-cerous chloride by intragastric dosing to suckling, juvenile and adult rats and measured whole body and gut radioactivity periodically. In the 2 studies, the authors observed that the whole-body retention of cerium chloride by suckling rats was greater than the retention by weanling and adult rats. Shiraishi and Ichikawa (1972) measured a decrease in retention of whole-body radioactivity among suckling rats dosed at 0, 7, and 14 days after birth by approximately 11, 6.5, and 1.5% of the administered dose, respectively, while a rapid decrease of the whole-body radioactivity through 10 days after dosing was observed in weanling and adult rat (2 weeks after dosing, a whole-body radioactivity of 0.08 and 0.018% of the administered dose was measured in the 21- and 100-day-old adults rats, respectively. In the study of Kostial et al. (1989b), whole-body radioactivity 6 days post-exposure in 2-week-old suckling rats orally dosed with [141Ce] was 40% of the administered dose. However, whole-body radioactivity 6 days post-exposure in adult (6–8-week-old) rats was only 0.05%. In both studies, the GI tract was found to be the main site for cerium chloride accumulation in both, suckling and adult rats.
Further, Stineman and colleagues (1978) reported that intragastric administration of[144Ce]-cerous chloride(in the presence of citrate) at doses of 1,000 mg/kg and 1,163 mg/kg in 6-8 week-old male Swiss ICR mice resulted in accumulation of cerium (97 to 99 %) in the stomach and duodenum and minimal accumulation in other organs. The highest levels in all organs were observed 4 hours after exposure and decreased over the 7-day observation period. Decreased levels in the stomach and duodenum did not appear to be associated with a corresponding increase in other organs, suggesting that cerium was excreted rather than distributed to the body.
Higher GI absorption according to age was also reported by Eisele et al. (1980) who gave single gavage doses of either [144Ce]-cerous chloride or [144Ce]-cerous citrate (concentration unreported) to 0–6- or 6–24-hour-old C3H mice and Sprague-Dawley rats and to 6–24-hour-old Yorkshire piglets. Radioactivity levels were measured in the GI tract and other tissues, including the remaining carcass, up to 21 days after exposure. In the 0–6-hour-old mice, a high of 31% of the administered dose of cerium chloride was retained in the body 9 days following exposure. At 21 days post administration, the amount of pooled cerium citrate and chloride retained was approximately 25%. The mice dosed 0–6 hours after birth retained more cerium in the GI tract throughout the 21-day observation period than the mice dosed 6–24 hours after birth. The 0–6- and 6–24-hour-old rats exhibited absorption of 9–10% of administered cerium 21 days after exposure. In the Yorkshire piglets, the absorbed dose did not differ significantly over the 21-day observation period.
Yorkshire piglets treated with [144Ce]-cerous chloride by gavage on the 1st or 4th day after birth and sacrificed from 4 or 18 or 3 or 21 days, respectively, after dosing absorbed 2.5–8% ofthe administered dose (Mraz and Eisele, 1977).Absorption was threefold greater in piglets treated at 1 day of age versus those treated at 4 days of age. Body content of cerium chloride did not differ significantly between piglets sacrificed at the earlier dates and those sacrificed later, indicating that absorption was almost complete within 3–4 days.
According to the above reported studies, in young (suckling) animals, soluble cerium appears to be retained in intestinal cells, particularly in the ileum, possibly resulting in a much greater absorption than in adult animals. However, cerium retained in intestinal cells has been demonstrated to be unavailable systemically (Inaba and Lengemann, 1972). The high gut retention of cerium in young animals may be associated with high pinocytotic activity of the newborn intestinal cells (Kostial et al., 1989b). Shiraishi and Ichikawa, (1972) and Kargacin and Landeka, (1990) who used pre-treatment with glucocorticoids (which cause precocious gut closure -decreased pinocytosis- and maturation of the metal absorptive process), before cerium oral administration to suckling rats, observed a drastic reduction in the amount of cerium chloride detected in gut tissue or a more rapid loss of an oral dose to feces. This finding suggests that the pinocytotic activity of the intestinal cells contributed to the differences.
It can be concluded from all the above reported studies, that solublecerium compounds are poorly absorbed following oral exposure. Observed absorption of soluble radioactive cerium salts (nitrate and chloride) from the GI tract of adult rats was found to be < to 0.1 % of the administered dose. Higher retention in the gastrointestinal (GI) tissues has been observed in suckling animals.However, cerium retained in intestinal cells of younger animals has been demonstrated to be unavailable systemically.
Therefore, the systemic availability after oral exposure of cerium oxide, that israther insoluble in water as illustrated by its very low water solubility (< 0.123μg/L at 20°C and at pH between 6.01 and 6.41, Weissenfeld M, 2007, K1), and for which no Kow can be determined (inorganic substance),cannot be greater than the soluble species investigated above and thus is considered as negligible.
The results of the toxicological studies on bulk cerium dioxide (included in the IUCLID dossier) are consistent with the assumption of a no or negligible oral absorption:
Following a single administration by oral route (at up to the limit dose of 5000 mg/kg)no mortality occurred and no relevant systemic clinical sign or changes in body weight was observed(Monnot, 1983, K1; Lambert et al., 1993, K2, Kumari et al. 2014a, K2).
Following repeated dose administration by the oral route at doses up to the limit dose of 1000 mg bulk CeO2/kg for up to approximately 42 days in male rats and 54 days in female rats in an OECD 422 study (Davies, 2010, K1), there was no relevant sign of toxicity in any of the parameters studied (including clinical signs, functional observational battery, body weight, food consumption, haematology or blood biochemistry), as well as in macroscopic and microscopic examinations. Further, no effect of the treatment was observed on reproductive parameters and performances as well as in the pups.Similarly, no significant systemic toxicological effects were also observed in an OECD 407 in which male and females rats were treated with bulk cerium dioxide at levels up to 600 mg/kg bw/day, the highest dose tested in this study (Kumari et al, 2014b, K3).
The absence of toxic effects in these studies indicates that the test substance and/or its degradation products or metabolites (if any) are not absorbed or are devoid of toxicity following oral dosing with cerium dioxide.
Based on the abovementioned information on the physicochemical characteristics and the available toxicological data on cerium dioxide and the indications from literature on the very limited absorptionafter oral exposure to cerium chloride and cerium nitrate (water soluble cerium compounds),the absorption factor after oral exposure to cerium dioxide was set at 0,1% as a worst case for risk assessment purposes.
· Respiratory absorption
Cerium has been detected from case reports in the lung tissue and alveolar macrophages of subjects believed to have been exposed, after prolonged occupational exposures, torare earth-containing material in the workplace. These workers weremainly exposed from fumes of carbon arc lamp (for photoengravers and movie projectionists) or dusts. Although the individuals described in these report cases had often concomitant exposure to other chemicals (e.g. carbon black, silicates, mixture of rare earths, etc.) and there were no information onprocedures of industrial hygiene in force and no quantification of exposure at the time of exposure in any of these studies, there data may provide some information on the lung deposition/retention and distribution of CeO2 in workers:
Transbronchial biopsies in a 60-year-old movie projectionist showed cerium concentrations of 11μg/g wet weight after 12 years of exposure (Porru et al., 2001). Microscopic examination of the tracheobronchial lymph nodes of a movie projectionist of 25 years revealed also grey granules in large macrophages, which were characterized as calcium and rare earth elements (cerium, lanthanum, and neodymium) by energy-dispersive analysis of X-rays (Waring and Watling, 1990). Similarly, McDonald et al. (1995) observed, by using a scanning electron microscope, particulate material (diameter range from <1μm to 5–10μm) localized within lung biopsy cellsin a patient with a history of optical lens grinding for 35 years.
Pairon et al. (1994) performed a retrospective evaluation of retention of cerium-containing particles in the lungs of workers previously exposed to mineral dusts. Broncho-alveolar lavage and lung tissue samples from mineral dust exposed workers and controls were examined for cerium content. In the seven cases that were judged to have high cerium particle retention (as defined by having lavage fluid or tissue cerium concentrations at least 5 times higher than those of controls), time since last exposure ranged from present to 29 years, with one patient’s exposure time not available. Further, aanalysis of broncho-alveolar lavage fluids performed by the same group, revealed cerium and phosphorus in the alveolar macrophages from a 58-year-old patient exposed to rare earth dusts and asbestos (Pairon et al., 1995). The cerium particles accounted for 70% of the particles observed in the lung tissue and were also identified in the interstitial macrophages.
The concentration of cerium in the lung and lymph nodes of subject exposed to smoke from cored carbon arc lamps for 46 years as a photoengraver was 167 and 5 μg/g wet tissue weight, respectively. Lung tissues were found to have cerium concentrations 2,800–207,000 times higher than those of urine, blood, or nails, suggesting that cerium particles in the lung are poorly mobilized (Pietra et al., 1985, Vocaturo et al., 1983; Sabbioni et al., 1982).
Thesecase reports provide support for the limited absorption of cerium deposited in the lung following inhalation exposure (IRIS-EPA, 2009).
Cerium compounds are classified into three categories according to their estimated rate of clearance from the respiratory tract in humans (derived from modeling animal data): Oxides and hydroxides of cerium, which are poorly soluble in body fluids, are in the slow-clearance category (years) and only 1% of the deposited particles is expected to be absorbed into the blood from the respiratory tract; nitrates, phosphates, and chlorides, with intermediate solubility, are in the intermediate-clearance category (weeks) and 5% to 10% of the deposited particles is expected to be absorbed into the blood from the nasopharyngeal region and 50% from the tracheobronchial region; sulfates and sulfides, which are readily soluble, are in the rapid-clearance category (days) (HEI report, 2001). Consequently,considering the impact of the solubility on the clearance and distribution of the material into the body, only animal studies that used poorly soluble forms of cerium were used in this assessment. All the studies reported below have used radioactive [144Ce] forms of cerium oxide or cerium hydroxide.
Lundgrenet al. (1992) exposed adult F344 rats to144Ce oxide aerosol for 5–50 minutes either once or repeatedly using a whole body exposure system. Particle activity median aerodynamic diameter ranged from 0.9 to 2.2 µm with geometric standard deviations ranging from 1.4 to 2.0. Animal were sacrificed 1 hour and from 3 to 672 days after exposure. Clearance was measured by whole body counts. Approximately 90 % of the initial whole-body burden of144Ce oxide was cleared within 7 days after exposure. Of the remaining initial whole-body burden, 7,1 +/- 4 % cleared with a half-time of about 32 +/- 1,5 days, and 1,2 +/- 0,07 % cleared with half-time of about 170 +/- 4,3 days under both experimental conditions (single or repeated exposures). A majority (56 to 90 %) of the initial lung burden in rats exposed once were cleared from the lungs with half-time of 5.7 to 42 days, while a lesser amount (10 to 41 %) was cleared with half-time of 36 to 180 days. The authors concluded that the clearance of the initial whole-body burdens of144Ce from rats exposed by inhalation to aerosols144Ce oxide was typical of that in rodents exposed to relatively insoluble aerosols. A major portion of the initial whole-body burden, representing primarily144Ce deposited in the upper respiratory tract and external contaminations, was cleared rapidly via gastrointestinal tract due to both mucociliary clearance from the upper respiratory tract and from grooming of the pellet.
Rapidclearance of [144Ce] was also observed in mice C57BL/6J that were nose only-exposed to144Ce oxide aerosols[MMAD 1.35–1.42μm] for 20 min to an unspecified concentration and observed for lifespan (Lundgrenet al. (1974). Eighty to 90 % of the initial body burden was cleared from the upper respiratory tract and large airways between days 0 and 6. Majority of body burden at various killing times between days 101 and 454 was in the lung (from 95%–84%).
More recently, Geraets L et al. (2012; K2) investigated absorption, tissue distribution, accumulation, and elimination of cerium dioxide (bulk and nano-CeO2) during and after a 28-day inhalation study in rats performed according to OECD guideline 412. Only results of bulk CeO2 (NM-213) are reported in this summary. Male Wistar rats were exposed to 55 mg/m3 CeO2 (particle concentration: 0.68 x 106 particles/cm3; MMAD: 1.40 ± 0.11 µm; CMD: 0.34 ± 0.06 µm) via inhalation route (nose-only), 6 h/day for 1 to 28 days with a number of exposures ranging from 1 to 20. At different time points during and after the study, tissue and blood samples were collected and analyzed for CeO2 content using ICP-MS and AES.
After both single and repeated exposures, the highest levels of CeO2 were found in lung tissues. After single exposure, 12.2 % of the inhaled dose was deposited in lung tissue. The estimation by a multiple path particle dosimetry model (MPPD) found 7 %. No cerium was detected in the blood of either control or treated rats, suggesting either a fast distribution from blood to tissue or a poor translocation of CeO2 to extrapulmonary tissues.
The available toxicological data are consistent with a limited absorption of bulk cerium dioxide after inhalation exposure:
In 2 acute inhalation studies performed according to OECD guideline 403, rats were exposed 4 hours to 2.01 mg/l (maximum technically administrable concentration) or to 5.05 mg/l bulk CeO2 and observed 14 days after exposure (Traynard J, 1983 (K1); Duchosal F, 1993 (K1), respectively). No mortality and no significant changes in body weight were seen when compared to controls in both study. Transient clinical signs (labored breathing for up to 24 hours after exposure) were observed in 1 animal exposed to 5.05 mg/l. At necropsy, the lungs of all animals in this study were incompletely collapsed with diffuse whitish foci that may suggest CeO2 deposition into the lung.
In a repeated dose toxicity inhalation study, male and female rats were exposed (nose only) to 0, 5, 50.5 and 507.5 mg/m3(MMAD = 1.8-2.2 µm) 6h/day, 5 days/week for 13 weeks in a study performed according to OECD guideline 413 (Viau, 1994; K1). Observations and measurements included mortality, clinical signs, body weight, food consumption, Functional Observational Battery, motor activity, hematology, clinical biochemistry, urinalysis, ophthalmological examination, gross pathological examination, organ weights and histopathological examination of selected tissues.
No treatment-related deaths or clinical signs occurred during the study. There were no overt systemic toxicological effects of treatments on body weight, food consumption, ophthalmology, clinical chemistry, and urinalysis. No behavioural changes and no significant differences in motor activity were observed in treated groups in the Functional Observational Battery assessment.
Treatment-related changes included changes in few hematological parameters (elevated segmental neutrophil counts) and in some organs weights (increase of lung and spleen weights). Macroscopic analyses showed no relevant findings except for lung and lymph nodes: discoloration or pale areas/foci and uncollapsed parenchyma in the lungs were observed in males and/or females at 50.5 mg/m3 and above. Enlargement or pale discoloration of the bronchial, mediastinal and pancreatic lymph nodes were also observed in all treated groups. At microscopic examination, no relevant findings were observed in the organs observed except pigmented material accumulation in the respiratory tract, liver and spleen and in bronchial, mandibular and mediastinal or pancreatic lymph nodes, as well as alveolar epithelial/lymphoid hyperplasia in the lungs and in lymph nodes and metaplasia in larynx, correlating with the presence of pigment in these tissues in a dose-response relationship.
From the results described above, in which quite high concentrations of bulk CeO2 (up to 507 mg/m3) were used, no relevant systemic effects specific to cerium dioxide as such were evidenced in this study but loco-regional "portal-of-entry" effects were observed.
Similar results were observed by Gosens I. et al (2014; K2) who exposed rats by inhalation (nose only) in a 28 days study performed according to the OECD guideline 412 with some deviations. Male and females rats were exposed 40 min to 6 h/day for 5 days/week during 4 weeks to 0, 5.9, 18.4 and or 55 mg/m3 of bulk CeO2 (NM-213). At the end of the exposure period, some animals from control (0) and high dose groups, were kept for a recovery period of 28 days before sacrifice. Clinical signs, mortality, body weight, and food consumption were monitored during the study and haematological parameters, clinical chemistry in blood and broncho-alveolar lavage fluid (BALF), inflammatory cell counts in BALF, urinalysis, fibrinolysis, and gross/histo-pathology were carried out at the end of the study to determine the local (pulmonary) and systemic effects of the CeO2 particles.
There were no exposure-related signs of toxicity, behaviour effects or mortality during the 4-week exposure period and recovery period. There was no evidence of systemic toxicity or haematological effects or organ weight changes. There was no macroscopic or microscopic change in the liver induced by bulk CeO2. However, macroscopic and microscopic finding (pale tracheobronchial lymph nodes, aggregates of brown/green particles and/or macrophages containing these particles were observed in the lungs, the trachea and the draining (tracheobronchial) lymph nodes at the end of the exposure period or in the recovery groups exposed to the high dose of CeO2. Increase in lung weights were also found directly after the end of exposure in female of the middle and high dose groups and in male from high dose recovery group.
Broncho-alveolar analyses showed a statistically significant increase in total cell counts in BALF, in neutrophils, macrophages and lymphocyte numbers, mainly in female high dose group that tend to decrease during the recovery period. Clinical/biochemical parameters measured in BALF (ALP, GGT, LDH, NAG, protein) were also significantly increases in males and females after bulk CeO2 exposures and after the 28-day recovery period though a noticeable decrease was observed for all parameters.
According to the authors, bulk CeO2 induce a dose-dependent pulmonary inflammation, but without any gross or histopathological changes immediately after exposure. The Inflammation was still present after the 28-day recovery period, albeit at a lower level. However, there was no evidence of systemic toxicity or other haematological changes and no macroscopic or microscopic change in the liver following exposure to any of the 3 tested CeO2. According to the authors, these data suggested that the adversity of the effects could be classified as minimal.
From the results reported above, it can be concluded that around 80 to 90 % of the inhaled bulk cerium dioxide will be rapidly cleared from the body within 0 to 7 days and the remaining fraction deposited into the lung will clear more slowly(several days/months) (Lundgren et al., 1992, 1974; Geraets et al., 2012). Cerium deposited in the alveolar region can be cleared to the gastrointestinal tract via mucociliary movement and thus eliminated via feces, or to the lymph nodes, or absorbed into the systemic circulation (HEI report, 2001). A portion may remain in the lung for year as seen in the case reports reported above. The studies of Viau et al (1994) and Gosens et al (2014) suggest a low systemic absorption since no systemic toxicity was observed in these study and no lesions of organs (excluding respiratory tract and associated lymph nodes), except pigmented material accumulation in liver and spleen were seen. However, none of the studies reported above estimated the exact absorbed fraction of CeO2.
Based on the abovementioned information and in absence of measured value of absorbed CeO2 fractions, if it is considered that almost all of the deposited lung fraction is totally absorbed,the absorption factor after inhalation exposure to cerium dioxide is set at 10 % as a worst case for risk assessment purposes.
- Dermal absorption
As no specific study on dermal absorption was identified for bulk CeO2, data from cerium chloride were used as a surrogate. Due to the higher solubility of the chlorides compared to the oxides the dermal absorption of the chloride is a worst-case estimate for the oxide. Further, in vitro data from nano CeO2, that, due to their smaller size, may show enhanced penetration, was also used in this assessment as a worst case to further assess the bulk CeO2 dermal penetration.
Inaba and Suzuki-Yasumoto, (1979)studied the uptake of radiolabled Cerium chloride (144Ce) through stripped and intact guinea pig skin during a 3 hour exposure period. The uptake through intact skin was negligible (<= 0.001%) while from stripped skin ca. 4% of the radioactivity was absorbed. The study demonstrated that absorption of a soluble cerium (III) salt, cerium trichloride through intact guinea pig skin is very low (<= 0.001%).
Mauro M. et al, (2019, K2) performed an in vitro investigation of the permeation of in-house synthesized 17-nm CeO2 NPs dispersed in synthetic sweat (1 g/L) using excised human skin on Franz cells. The study was performed according to OECD test guideline n° 428. Experiments were performed using intact and needle-abraded skin, separately. In both experiments, the exposure chambers of three Franz diffusion cells were filled an amount of CeO2 of 0.6 mg/cm2. After 24 h, the receiving solutions and the skin pieces were analyzed using ICP-OES and electron microscopy methods.
After 24 hours, the average amount of Ce into intact and damaged whole skin samples was 3.64 +/- 0.15 and 7.07 +/- 0.78 µg/cm2, respectively. Ce concentration in the receiving solution was 2.0 +/-0.4 (as in the blank cells) and 3.3 +/- 0.7 ng/cm2 after 24 h. The Ce content was higher in dermal layers of damaged skin compared to intact skin (2.93 +/- 0.71 µg/cm2 and 0.39 +/- 0.16 µg/cm2, respectively).
The authors concluded that nanoCeO2 could not permeate intact skin after prolonged exposure (24 hours), but this permeation was possible - if at a low level - using an abraded skin protocol. They further suggested that this behavior is probably due to the very low ionization of these metal oxides in synthetic sweat, resulting in a small concentration of free metal ions in the donor phase being able to cross over the physiological barriers.
As an illustration, in a study performed according to OECD 402, following acute exposure of rats to a dermal dose of 2000 bulk CeO2 mg/kg and observation up to 14 days following application (Monnot G., 1983, K1), no noteworthy systemic sign (other than slightly reduced activity on day 1, a non-specific sign considered a result of the procedure) was observed, supporting the lack of absorption through the skin. Furthermore, the bulk CeO2 is devoid of skin and eye irritation potential that could have enhanced dermal penetration (Gonnet JF and Guillot GP., 1983; Lambert CE. 1993; Park B et al., 2007).
Based on the abovementioned information on the very limited absorptionafter dermal exposure to cerium trichloride (a water soluble cerium compound), the absence/negligible permeation of nanoCeO2 into the skin in in vitro models and the absence of systemic toxicity after exposure by dermal route to bulk cerium dioxide,the absorption factor after dermal exposure to cerium dioxide is considered as negligible and was set at 0,1% as a worst case for risk assessment purposes.
DISTRIBUTION
- After oral exposure:
There is no data that give information on the distribution of cerium dioxide or other insoluble form of cerium after exposure by oral route. However, few studies, that all used the soluble cerium chloride, that may be absorbed more easily systematically, provided some information on the distribution of cerium after oral exposure:
Stineman and colleagues (1978) reported that intragastric administration of cerium chloride (in the presence of citrate) at doses of 1,000 mg/kg and 1,163 mg/kg in mice resulted in accumulation of cerium in the stomach and duodenum and minimal accumulation in other organs. The highest levels in all organs were observed 4 hours after exposure and decreased over the 7-day observation period. Decreased levels in the stomach and duodenum did not appear to be associated with a corresponding increase in other organs, suggesting that cerium was excreted rather than distributed to the body.
Several authors (Kostialet al., 1989b; Inaba and Lengemann, 1972; Shiraishi and Ichikawa, 1972, Durbinet al., 1956); see the above section “Absorption”) who used a radioactive soluble (chloride or nitrate) form of cerium in their experiments, suggested that the gastrointestinal tract of newborn rodents may have higher and more prolongedretention or may be more permeable to ingested cerium than that of older animals. As an example, Inaba and Lengemann (1972) observed that weaned pups excreted cerium rapidly via feces after exposure to a single dose of 141Ce nitrate (dose not specified): only 0.04% of the administered dose remained in the body 3 days after dosing. However, suckling pups lost radioactivity at a slower rate with newborn pups having the lowest rate of loss. For pups at age 0 days, 0.34% of the administered dose was calculated to be in body tissues (excluding the gastrointestinal tract) after 1 day, 2.1% after 16 days, and 2.5% after 24 days. The increased tissue retention was associated with increased uptake of cerium by intestinal epithelial cells,likely in the vacuoles, which eventually are sloughed off into the intestinal lumen. Retention was also affected by the type of diet. Pups whose diet was changed to commercial food at 17 days of age lost radioactivity faster than pups of a similar age still suckling, and 43-day-old rats fed dried whole milk had a much slower loss than rats of the same age on a commercial grain diet.
Using 144Ce chloride by oral gavage in rats, Shiraishi and Ichikawa (1972) observed that, although the cerium distribution pattern was almost identical in suckling and adult rats (100 days old), the tissue concentration in bone, liver, spleen, and kidney was 2 to 3 orders of magnitude higher in suckling rats. Most of the retained radioactivity was found in the skeleton followed by the liver.
Following 6 and 12 weeks of oral exposure to dietary concentrations of 20 or 200 ppm cerium chloride (corresponding approximately to 2.6 and 26 mg Ce/kg/day), the concentration of cerium in the kidney, liver, lung, and spleen of male ICR mice was significantly elevated in the high dose group relative to controls, but no pathologic alterations were observed in these organs after microscopic observation (Kawagoeet al., 2005). The authors found that the lung and the spleen contained the highest cerium concentrations. However, pathological alterations were not detected in the kidney, liver, lung, or spleen by microscopic observation
- After Inhalation exposure:
Geraets L et al. (2012; K2) investigated absorption, tissue distribution, accumulation, and elimination of cerium dioxide (bulk and nano-CeO2) in a repeated dose toxicity study performed according to OECD guideline 412. Male rats were exposed to 55 mg/m3 bulk CeO2 (MMAD: 1.40 ± 0.11 µm) by inhalation (nose-only) 6 h/day for 1 to 28 days. At different time points during and after the study, tissue and blood samples were collected and analyzed for CeO2 content using ICP-MS and AES.
After both single and repeated exposures, the highest levels of CeO2 were found in lung tissues. After single exposure, 12.2 % of the inhaled dose was deposited in lung tissue. No cerium was detected in the blood of either control or treated rats, suggesting either a fast distribution from blood to tissue or a poor translocation of CeO2 to extrapulmonary tissues. Distribution of the inhaled CeO2 particles to various extrapulmonary tissues was also observed, but largely in a lower extent (µg/kg tissue) than in the pulmonary tissues (mg/kg tissue).CeO2 detected in the extrapulmonary tissues after a single exposure was less than 0.2% of the inhaled dose. Indeed, after a single 6-h exposure, CeO2 sample was also distributed to extrapulmonary tissues with an order of concentration of testicles < kidney < liver < spleen < brain < epididymis. Furthermore, tissue clearance of the particles seemed to be slow, and insignificants amounts of CeO2 (estimated based only on CeO2 contents in the investigated tissues) were eliminated within the 48-72 h following the end of exposure period. Repeated exposure to CeO2 was found to result in significant increasing CeO2 concentrations in lungs and liver, indicating that accumulation occurred in these tissues.
Thomas et al (1972) exposed 4-month old Holtzman rats whole body to144Ce hydroxide aerosol (MMAD 1,4 µm) for 25 minutes to low and high radiation doses (concentration not indicated).Animals were maintained until time of death (47–607 days after exposure). Deposition and distribution were measured using whole-body counts and counts in feces and urine (up to 120 days) and by organ counts at time of death. An average whole-body deposition of 28% of inhaled aerosol was found and no overt difference were seen at the two concentrations used. Initial rapid clearance of about 80% of initial body burden (IBB) was observed with fecal/urinary ratio of 50 decreasing to 10 by day 100. Radioactivity distributed to lung, liver, and skeleton varied with day of death. Example: lung burden relative to the initial body burden (IBB) was 3–8% (day 101–103) and 2.7–1.9% (day 230– 289), liver burden was 8–9% and 1–6%, and skeleton burden was 20–29% and 7–17% during same periods. Two animals surviving to day 528 and 607 had < 1% of IBB in lung and liver and 5% in skeleton. Radioactivity was also detected in kidney and spleen.
Lundgrenet al. (1992) exposed adult F344/Crl rats to radiolabelled-cerium oxide aerosol for 5–50 minutes or to bimonthly exposures of 25 minutes for 1 year; rats were sacrificed at 1 hour and 3, 7, 14, 28, 56, 112, 224, 448, 560, and 672 days after exposure. The lungs, heart, liver, spleen, kidney, and skeleton (remaining carcass) were measured for cerium. Cerium was detected in the liver and skeletonin increasing percentages of body burden with respect to time, while cerium was not detected in the spleen and kidneys.
In a repeated dose toxicity inhalation study performed by Viau, (1994; K1; OECD 413) in which, male and female rats were exposed (nose only) to 0, 5, 50.5 and 507.5 mg/m3(MMAD = 1.8-2.2 µm) 6h/day, 5 days/week for 13 weeks, no overt effects of treatments were found on body weight, food consumption, ophthalmology, clinical chemistry, and urinalysis and no behavioural changes and no significant differences in motor activity were observed in treated groups.Increase of lung and spleen weightswere noted and pigment accumulation was observed at histopathological examination in these organs as well as mandibular lymph nodes in the high-concentration group only (507 mg/m3) and in bronchial or mediastinal lymph nodes, nasal cavity and bronchi (in low-dose, mid-dose and high-dose groups), in trachea and pancreatic lymph nodes and in larynx (in mid-dose and high-dose groups). In the organs examined in this study, (adrenals, aorta, bone marrow, sternum, brain, cecum, colon, duodenum, epididymis, esophagus, eye, heart, ileum, jejunum, kidney, mesenteric lymph node, mammary gland, skeletal muscle , optic nerve, sciatic nerve, pancreas, parathyroid, pharynx, pituitary gland, prostate, salivary gland, seminal vesicle, skin, cervical spinal cord, stomach, testis, thymus, thyroid, tongue, urinary bladder, ovary, uterus), no relevant histopathological findings were noted.
These results suggest that bulk CeO2 was distributed to at least to lymph nodes, liver and spleen.
- After exposure via intravenous route
Although the intravenous route is not an expected route of exposure, various study results using this route were reported below to order to help in understanding of the distribution of cerium once it is in the body.
Several studies that used intravenous injections of a soluble form of cerium (chlorides) have shown that cerium disappears quickly from blood after injection:
Nakamura and colleagues (1997) found that about 70% of the initial dose of cerium chloride administered to rats (10 or 20 mg/kg) was found in serum 2 hours after administration and had almost entirely disappeared from the blood within 24 hours. At this time point, only 3% of the initial dose remained in the blood, while the majority of the dose was found inliver (70%), bone (12%), spleen (3%), lungs (1%) and kidneys (0,5 %) where it was retained for a long time. No substantial difference was observed in the distribution pattern at the two dose levels.
Bjondahl (1976) who injected 5.5 mg/kg of [144Ce] chloride in mice also showed that one day after injection, less than 1% of the initial dose was present in serum, but a concomitant accumulation of cerium was noted in the liver (4,5 % of the initial dose). Excretion through the feces (3 % of the initial dose by day 3) was slow and was still noticeable 14 days after injection.
Similar results were found by Arvela and collaborators (1991) in a study in which mice were injected with 0,5 to 2 mg/kg of [144Ce] chloride. They showed that the major organs accumulating intravenously injected cerium chloride (0.5 to 2 mg/kg) were the liver and spleen.
From the results reported above, it can be concluded that, once absorbed, cerium compounds seem to be distributed mainly in liver, skeleton and to a lesser extent spleen and kidney whatever the route of exposure.
METABOLISM
As an element, cerium is neither created nor destroyed within the body. The cerium compounds (e.g., cerium chloride, cerium oxide) may be altered as a result of various chemical reactions within the body, particularly dissolution, but data have not demonstrated a change in the oxidation state of the cerium cation (IRIS-EPA, 2009).
Berry and coworkers (1988, 1989, 1997), who have examined the intracellular behavior of soluble (chloride) and insoluble (oxide) cerium aerosols in rats, found that cerium becomes concentrated and precipitates as insoluble phosphates in lysosomes of alveolar macrophages after inhalation that could be linked with the high levels of phosphates in the lysosomes due to the action of phosphatases that release phosphate from substrates.The authors suggested that the precipitation and concentration of cerium within the lysosomes may serve to inhibit diffusion of the metal to other tissues.
No microscopic finding in the major metabolizing tissues (liver, kidneys) illustrative of metabolic activity were seen following repeated dose administration by the oral route at doses up to the limit dose of 1000 mg/kg for up to approximately 42 days in male rats and 54 days in female rats or by inhalation (nose only) at concentrations up to 507 mg/m3 for 13 weeks in rats (Davies R, 2010, K1; Viau A., 1994, K1).These results do not suggest a transformation of bulk CeO2 to more hazardous forms in the liver or kidney.This is also supported by the negative results observed inin vitromutagenicity (Ames test: McVety, 2007, K1; Shimizu et al., 1985, K1; Park et al., 2007, K2 - In vitro gene mutation study in mammalian cells: Wollny, 2006, K1), both in the presence or absence of exogenous metabolic activation system.
ELIMINATION
No quantitative estimate of elimination of cerium compounds in faeces and urine were identified. However, it appear from the studies reported in this assessment that almost all (> 99, 9 %) the cerium (soluble forms) administrated by oral route in adult animals was rapidly eliminated from the body, most probably via faeces and under an unmodified form (Shiraishi and Ichikawa, 1972; Kargacin and Landeka, 1990;Inaba and Lengemann, 1972; Stineman et al., 1978).Nevertheless, elimination of orally administered soluble cerium appeared to be age dependent in animals, with suckling animals absorbing higher cerium into the GI tissues. Cerium remained in the intestinal cells and may be minimally available systemically and is eventually eliminated in the faeces as the intestinal cells die and are replaced (Shiraishi and Ichikawa, 1972; Kargacin and Landeka, 1990; Kostial et al., 1989; Inaba and Lengemann, 1972). Insoluble forms of cerium such as cerium dioxide are expected to be eliminated in the same way and in similar order of magnitude if not in higher quantity due to its insolubility.
After inhalation exposure, cerium is also eliminated via faeces but some amounts can be eliminated via urine (Thomas et al., 1972).An initial short-term clearance rates ranging from ~ 75 to 95 %, depending on the species tested and length of clearance time investigated, were observed with insoluble cerium compounds (oxide and hydroxide) (Boecker and Cuddihy, 1974; Lundgren et al., 1992; Kanapilly and Luna, 1975; Thomas et al., 1972).This rapid elimination from the body is also due to transport up the respiratory tract by the mucociliary escalator and eventual swallowing of the material, that will finally be eliminated via feces (Lundgren et al. 1992).
Following a single administration by oral route (at the limit dose of 5000 mg/kg), whitish discoloration of the faeces was observed, likely indicative of faecal elimination of the test substance as unchanged material (Monnot, 1983, K1).
REFERENCES:
Arvela P, Kraul H, Stenbäck F, Pelkonen O. (1991). The cerium-induced liver injury and oxidative drug metabolism in DBA/2 and C57BL/6 mice. Toxicology 69:1–9.
Berry, JP; Meignan, M; Escaig, F; et al.(1988) Inhaled soluble aerosols insolubilised by lysosomes of alveolar cells. Application to some toxic compounds; electron microprobe and ion microprobe studies. Toxicology 52(1–2):127–139.
Berry, JP; Masse, R; Escaig, F; et al.(1989) Intracellular localization of cerium. A microanalytical study using an electron microprobe and ionic microanalysis. Hum Toxicol 8(6):511–520.
Berry JP, Zhang L, Galle P, Ansoborlo E, Hengé-Napoli MH, Donnadieu-Claraz M. (1997).Role of alveolar macrophage lysosomes in metal detoxification. Microsc Res Tech 36:313–323.
Bjondahl K. (1976). Differences in liver weight, mortality in cerium-treated mice and 144Ce levels in blood, liver, urine, and faeces at various intervals after treatment with nafenopin and pregnenolone 16-α-carbonitrile (PCN). Med Biol 54:454–460.
Boecker, BB; Cuddihy, RG. (1974) Toxicity of 144Ce Inhaled as 144CeCl3 by the beagle: metabolism and dosimetry. Radiat Res 60(1):133–154.
Davies R (2010) Combined repeated dose study with the reproduction/developmental toxicity screening test by oral route (gavage) in rats. CIT, Evreux, France. Report no. 33179 RSR. Unpublished report.
Duchosal F. (1993) 4-hour Acute Inhalation Toxicity Study with Oxyde de Cérium (Opaline) in Rats. RCC Ltd. Itingen, Switzerland. Report no. 330974. Unpublished report.
Durbin, PW; Williams, MH; Gee, M;et al.(1956) Metabolism of the lanthanons in the rat. Proc Soc Exper Biol Med 91:78–85.
Eisele GR, Mraz FR, Woody MC. (1980). Gastrointestinaluptake of 144Ce in the neonatal mouse, rat, and pig. Health Phys 39:185–192.
Geraets L, Oomen AG, Schroeter JD, Coleman VA, Cassee FR (2012). Tissue Distribution of Inhaled Micro- and Nano-sized Cerium Oxide Particles in Rats: Results from a 28-day. Exposure Study Toxicol Sci., 127(2): 463-73.
Gonnet JF, Guillot GP (1983). Oxyde de Cerium: Tests de tolérance locale chez le lapin (indice d'irritation primaire cutanée et indice d'irritation oculaire) et Test de sensibilitation cutanée chez le cobaye.Institut Français de Toxicologie, Les Oncins, Saint-Germain-sur-l'Arbresle, France.Report no. 302314. Unpublished report.
Gosens I, Mathijssen LEA, Bokkers BGH, Muijser H, Cassee FR.(2014). Comparative hazard identification of nano- and micro-sized cerium oxide particles- b ased on 28-day inhalation studies in rats. Nanotoxicology 8(6):643-53.
HEI (Health Effects Institute). (2001) Evaluation of human health risk from cerium added to diesel fuel. HEI communication 9. North Andover, MA; Flagship Press. Available online at http://pubs.healtheffects.org/.
Inaba, J; Lengemann, FW. (1972) Intestinal uptake and whole-body retention of 141Ce by suckling rats. Health Phys 22:169–175.
Inaba, J. and Suzuki-Yasumoto, M.S. (1979). A kinetic study of radionuclide absorption through damaged and undamaged skin of the guinea pig. Health Phy 37:592-595.
IRIS-EPA (2009). Toxicological Review of Cerium Oxide and Cerium Compounds (Vol. 2). US Environmental Protection Agency EPA/635/R-08.
Kanapilly, GM, Luna, RJ. (1975) Deposition and retention of inhaled condensation aerosols of144CeO2 in Syrian hamsters. In: Boecker, BB; Rupprecht, FC; eds. Annual report of the Inhalation Toxicology Research Institute.
Kargacin, B; Landeka, M. (1990) Effect of glucocorticoids on metal retention in rats. Bull Environ Contam Toxicol 45(5):655–661.
Kawagoe, M; Hirasawa, F; Wang, SC; et al. (2005) Orally administered rare earth element cerium induces metallothionein synthesis and increases glutathione in the mouse liver. Life Sci 77:922–937.
Kostial, K; Kargacin, B; Blanusa, M;et al.(1989) Location of mercury, cerium, and cadmium in the gut of suckling and weaned rats. Period Biol 91(3):321–326.
Kumari M, Kumari SI, Kamal SSK, Grover P (2014a).Genotoxicity assessment of cerium oxide nanoparticles in female Wistar rats after acute oral exposure.Mutat Res Genet Toxicol Environ Mutagen., 775-776:7-19.
Kumari M, Kumari SI, Grover P (2014b).Genotoxicity analysis of cerium oxide micro and nanoparticles in Wistar rats after 28 days of repeated oral administration.Mutagenesis., 29(6):467-79
Lambert CE, Barnum EC, and Shapiro R (1993) Acute Toxicological Evaluation of Cerium Oxide Journal of the American College of Toxicology, 12(6): 612-33, 1993
Lundgren DL, McClellan RO, Thomas RL, Hahn FF, Sanchez A. (1974). Toxicity of inhaled 144CeO2 in mice. Radiat Res 58:448–461.
Lundgren, D. L., Hahn, F. F., Diel, J. H., & Snipes, M. B. (1992).Repeated Inhalation Exposure of Rats to Aerosols of: I. Lung, Liver, and Skeletal Dosimetry. Radiation research, 132(3), 312-324.
Mauro M, Crosera M, Monai M, Montini T, Fornasiero P, Bovenzi M, Adami G, Turco G and Larese Filon F (2019). Cerium Oxide Nanoparticles Absorption through Intact and Damaged Human Skin. Molecules 2019, 24, 3759.
McDonald JW, Ghio AJ, Sheehan CE, Berhnardt PF, RoggliVL. (1995). Rare earth (cerium oxide) pneumoconiosis: Analyticalscanning electron microscopy and literature review.Mod Pathol 8:859–865.
McVety S. (2007) Cerium Oxide - Bacterial Mutation Test. Charles RIver Laboratories Preclinical Services Montreal Inc. Transcanadienne Senneville, CANADA.Study no. 961490. GLP. Unpublished report.
Monnot G. (1983) Oxyde de Cérium (Opaline broyage spécial n°5) - Essais de toxicité aiguë par voies orale et percutanée chez le rat. Institut Français de Toxicologie, Saint-Germain-sur-l'Arbresle, France. Study no. 301229. Not GLP. Unpublished report.
Mraz, FR; Eisele, GR. (1977) Gastrointestinal absorption and distribution of 144Ce in the suckling pig. Health Phys 33:494–495.
Nakamura Y, Tsumura Y, Tonogai Y, Shibata T, Ito Y. (1997).Differences in behavior among the chlorides of seven rare earth elements administered intravenously to rats. Fundam Appl Toxicol 37:106–116.
Pairon JC, Roos F, Iwatsubo Y, Janson X, Billon-Galland MA, Bignon J, Brochard P. (1994).Lung retention of cerium in humans.Occup Environ Med 51:195–199.
Pairon JC, Roos F, Sebastién P, Chamak B, Abd-Alsamad I, Bernaudin JF, Bignon J, Brochard P. (1995). Biopersistence of cerium in the human respiratory tract and ultrastructural findings. Am J Ind Med 27:349–358.
Park B, Martin P, Harris C, Guest R, Whittingham A, Jenkinson P, Handley J (2007). Initial in vitro screening approach to investigate the potential health and environmental hazards of EnviroxTM - a nanoparticulate cerium oxide diesel fuel additive. Part Fibre Toxicol. 5(4): 12
Pietra, R; Sabbioni, E; Ubertalli, L; et al.(1985) Trace elements in tissues of a worker affected by rare-earth pneumoconiosis. A study carried out by neutron activation analysis. J Radioanal Nucl Chem 92(2):247–269.
Porru, S; Placidi, D; Quarta, C; et al.(2001) The potential role of rare earths in the pathogenesis of interstitial lung disease: a case report of movie projectionist as investigated by neutron activation analysis. J Trace Elem Med Biol 14(4):232–236.
Sabbioni E, Pietra R, Gaglione P, Vocaturo G, Coluombo F, Zanoni M, Rodi F. (1982). Long-term occupational risk of rare-earth pneumoconiosis. A case report as investigated by neutron activation analysis. Sci Total Environ 26:19–32.
Shimizu, H; Suzuki, Y; Takemura, N; et al.(1985).The results of microbial mutation test for forty-three industrial chemicals. Sangyo Igaku. Jpn J Ind Health 27:400–419.
Shiraishi, Y; Ichikawa, R. (1972) Absorption and retention of144Ce and 95Zr-95Nb in newborn, juvenile and adult rats. Health Phys 22:373–378
Stineman CH, Massaro EJ, Lown BA, Morganti JB,Al-Nakeeb S. (1978). Cerium tissue/organ distribution and alterations in open field and exploratory behavior following acute exposure of the mouse to cerium (citrate). J Environ Pathol Toxicol 2:553–570.
Traynard J, (1983).Cerium Oxide - Acute toxicity study by inhalation in the rat.Institut Français de Toxicologie, Les Oncins, Saint-Germain-sur-l'Arbresle, France.Report no. 302217. Unpublished report.
Thomas RL, Scott JK, Chiffelle TL. (1972). Metabolism and toxicity of inhaled 144Ce in rats. Radiation Res 49:589–610.
Viau A (1994) A 13-week inhalation toxicity and neurotoxicity study by nose-only exposure of a dry powder aerosol of Ceric Oxide in the albino rat. Bio-Research Laboratories Ltd. Montréal, Canada. Report no. 90831. Unpublished report.
Vocaturo G, Colombo F, Zanoni M, Rodi F, Sabbioni E, Pietra R. (1983). Human exposure to heavy metals: Rare earth pneumoconiosis in occupational workers. Chest 83:780–783.
Waring PM, Watling RJ. (1990). Rare earth deposits in a deceased movie projectionist: A new case of rare earth pneumoconiosis? Med J Aust 153:726–730.
Weissenfeld M, (2007). Determination of the melting point / melting range and the boiling point / boiling range of opaline de polissage (cerium oxide). RCC Ltd, Itingen, Switzerland. Report no. A17381.Unpublished report.
Wollny H-E (2006). Gene mutation assay in Chinese Hamster V79 cells in vitro (V79 / HPRT) with Opaline de polissage RCC-CCR, Rossdorf, Germany. Study no. 914700. GLP. Unpublishedreport.
Description of key information
Key value for chemical safety assessment
- Bioaccumulation potential:
- low bioaccumulation potential
- Absorption rate - oral (%):
- 0.1
- Absorption rate - dermal (%):
- 0.1
- Absorption rate - inhalation (%):
- 3
Additional information
The following basic toxicokinetic information could be extrapolated from the experimental toxicological data available on nanometric cerium dioxide (nano-CeO2), an insoluble inorganic substance (Tentschert et al., 2020; Andreescu D et al., 2014; Brunner TJ et al., 2006; He X et al., 2010; Keller J et al., 2014; Molina RM et al., 2014/Konduru NV et al., 2015; Van Hoecke K et al., 2009; Yokel RA et al., 2014). To date, more than 20 publications on nano-CeO2 toxicokinetic were considered; these studies were all conducted in murine models (i.e., rats or mice) but used different routes of administration (i.e., gavage, inhalation, dermal, intratracheal instillation (i.t.), intravenous (i.v.), intraperitoneal (i.p.), or intravitreal injection).
Almost all studies displayed a more or less detailed characterisation of nano-CeO2 physico-chemical properties. Among other things, authors attempted to characterise the agglomeration/aggregation state of nanoparticles. However, it has to be noted that there was no clear definition of the terms "agglomeration" and "aggregation" in any of the available studies, thus both terms should be considered as being used in an indistinguishable manner.
ORAL ROUTE (GAVAGE)
Six published studies are currently available on orally administered nano-CeO2 displaying various physico-chemical properties (He X et al., 2010; Hirst SM et al., 2011; Kumari M et al., 2014; Molina RM et al., 2014/Konduru NV et al., 2015; Park EJ et al., 2009). These in vivo studies were conducted in rats or mice acutely or repeatedly exposed to the nanoparticles by oral route.
In a first study, Molina RM et al. (2014)/Konduru NV et al., 2015 investigated the bioavailability, tissue distribution, clearance and excretion of radioactive 141cerium (141Ce) after gavage of neutron-activated 141nano-CeO2 in male rats. There was no mention to any guideline or good laboratory practices (GLP). However, the study was overall well documented and met the generally accepted scientific principles. Thus, the publication was awarded a reliability score of 2 (Klimisch, 1997) and was flagged as weight of evidence.
A commercial nano-CeO2 (NM-212) provided by Mercator GmbH (Molina et al, 2014) and an in-house synthesised (Konduru et al., 2015) were used in this study. Nano-CeO2 powder was neutron activated; the process generated the radioisotope 141Ce. The physico-chemical characterisation was performed on non-radioactive forms of nanoCeO2, thus supposing that radioactive nano-CeO2 had the same properties as its non-radiolabelled counterpart. The characterisation is detailed below in Table 1.
Table 1: Summary of the physico-chemical characteristics available on the various nano-CeO2 tested by oral route
Parameters |
He X et al. (2010) |
Hirst SM et al. (2011) |
Kumari M et al. (2014) |
Kumari M et al. (2014) |
|
- |
- |
Nano-CeO2 |
Bulk CeO2 |
Supplier |
None (in-house synth.) |
None (in-house synth.) |
Sigma Chemical Co. Ltd |
Sigma Chemical Co. Ltd |
Synthesis method |
Precipitation method |
Simple wet chemistry method |
No data |
No data |
Primary particle size |
6.6 ± 0.9 nm |
3 to 5 nm |
24.2 ± 1.63 nm |
3.14 ± 1.29 µm |
Particle size distribution |
12.8 ± 2.2 nm in aqueous solution |
10 to 50 nm |
191.2 ± 20.25 nm in Milli-Q water |
Not detectable |
Stability |
Agglomeration / aggregation |
Agglomeration |
Agglomeration |
No data |
Specific surface area |
86.85 m²/g |
No data |
No data |
No data |
Surface charge (zeta potential) |
+30.1 mV |
No data |
-17 mV in Milli-Q water at pH 7 |
Not detectable |
Isoelectric point |
No data |
No data |
No data |
No data |
Shape |
No data |
No data |
Polyhedron crystals |
Polyhedron crystals |
Crystallinity |
Crystalline (cubic, fluorite) |
Crystalline (fluorite) |
No data |
No data |
Purity / impurities |
No data |
No data |
99.95% purity |
99.9% purity |
Solubility |
Insoluble (ca. 0.12%) at neutral and acid pH |
No data |
No data |
No data |
Oxidation degree |
No data |
Mixing of Ce(III) and Ce(IV), with a relatively higher abundance of Ce(III) |
No data |
No data |
Surface properties |
No data |
No data |
No data |
No data |
pH of nano-CeO2 suspension |
No data |
≤ 3.5 |
No data |
No data |
Parameters |
Molina RM et al. (2014)/Konduru NV et al. (2015) |
Park EJ et al. (2009) |
|
|
|
NM-212 / in house synthsised |
- |
|
|
Supplier |
Mercator GmbH / none |
None (in-house synth.) |
|
|
Synthesis method |
No data / Flame spray pyrolysis |
High temperature - Supercritical method |
|
|
Primary particle size |
40 nm / 39.2 |
30 nm |
|
|
Particle size distribution |
207 to 432 nm in distilled water / 136 +/- 1 nm |
No data |
|
|
Stability |
Agglomeration (35 nm to 7 µm) / - |
No data |
|
|
Specific surface area |
27 / 28 m²/g |
No data |
|
|
Surface charge (zeta potential) |
+37 to +42 in water / +34.5 +/- 3.1 mV |
No data |
|
|
Isoelectric point |
> pH 10 (always cationic) in water / - |
No data |
|
|
Shape |
Roughly globular / - |
Cubic-like |
|
|
Crystallinity |
Crystalline (cubic, cerianite) / - |
Crystalline with a crystal size of 30 nm |
|
|
Purity / impurities |
ca. 99.3% purity / - |
No data |
|
|
Solubility |
0.7% organic contaminations (ester + alkyl groups) on the particle surface as a very thin and homogeneous layer around the pure inorganic particles / - |
No data |
|
|
Oxidation degree |
Insoluble in various media / - |
No data |
|
|
Surface properties |
Mainly as Ce(IV) but 14% as Ce(III) / - |
No data |
|
|
pH of nano-CeO2 suspension |
Surface chemistry composed of 79.9% C, 17.7% O and 2.4% Ce / - |
No data |
|
|
- : No data
The nanoparticles were virtually insoluble under all conditions tested: for 24 h in DMEM with 10% foetal calf serum (FCS), for 28 days in phosphate-buffered saline (PBS, to simulate the surface of epithelium), 28 days in phagolysosomal simulant fluid (PSF, to simulate the phagolysosomes of macrophages), 1 day in 0.1N HCl (to simulate the stomach environment following oral intake), or 7 days in a simulated intestinal fluid (fasted state, FaSSIF). Nano-CeO2 was also found to be photocatalytically active.
In both studies, male Wistar rats (at least 5/group) were exposed once to 0 (vehicle control: sterile distilled water) or 5 mg/kg of nano-CeO2 by gavage. All treated rats were sacrificed 5 min and 7 days post-administration. Then, the following tissue samples were collected: blood, plasma, serum, lung, bone marrow, femoral bone, skin, brain, skeletal muscle, testicle, kidneys, spleen, heart, liver, stomach, small intestine, large intestine, and caecum. Moreover, 24-h samples of faeces and urine were collected at selected time points (0–24 hours, 2–3 days and 6–7 days post-gavage). Cerium (Ce) content in the samples was determined by measuring the radioactivity (141Ce) using a gamma counter.
In both studies, five minutes after gavage, nearly 100% of 141nano-CeO2 dose (5 mg/kg) was recovered in the stomach of exposed rats. Very low levels of 141Ce from nanoparticles (0.003 to 0.004) were measured in all organs combined, examined at 7 days. Moreover, nearly the entire 141Ce dose from 141nano-CeO2 was rapidly excreted in the faeces (ca. 75% of the administered dose at day 1 post-gavage, ca. 90% at day 3, and ca. 95 -99% at day 7) and urine (< 0.005% of the administered dose) over 7 days.
The authors concluded that ingested nano-CeO2 posed almost no risk of absorption since ingested nano-CeO2 was almost entirely excreted in the faeces. Thus, the risk of Ce tissue accumulation and potential toxicity could be considered as very low and probably insignificant when nano-CeO2 is ingested. In conclusion, the tested nano-CeO2 displayed the classical behaviour of poorly soluble particles: low bioaccumulation and high faecal excretion.
Park EJ et al. (2009) also evaluated the tissue distribution of nano-CeO2 in rats exposed via oral route in an acute oral toxicity study performed according to Korean Food and Drug administration Guideline. There was no mention to GLP. The physico-chemical characteristics of nano-CeO2 and the description of test animals (e.g., age, weight, diet) were insufficiently documented. Moreover, there was no data available regarding the experimental validation of the detection method, inductively coupled plasma-mass spectrometry (e.g., limit of detection). Therefore, the study was awarded a reliability score of 3 (Klimisch, 1997) and was flagged as supporting study.
In-house synthesised nano-CeO2 was used in this study. The physico-chemical characteristics of these nanoparticles are displayed in Table 1 above.
Male Sprague-Dawley (SD) rats (4 to 6/group) were administered a single nano-CeO2 dose of 0 (control), 100 or 5000 mg/kg by gavage and sacrificed on day 1, 7 and 14 after exposure. Tissue distribution analysis in liver, kidney, spleen, lung, testicles, and brain was performed using inductively coupled plasma-mass spectrometry (ICP-MS).
When rats were treated with both doses of nano-CeO2 and were sacrificed on day 1, nano-CeO2 accumulation in rat tissues seemed to occur mainly in lungs at both doses and slightly in liver and kidney at 5000 mg/kg. However, the increase was only statistically significant for the lung tissue. Thus, according to the authors, the lungs seemed to be the main target organ of orally administered nano-CeO2. The elevated level was decreased on day 7 and day 14 in a time-dependent manner. In the low-dose treated group, the CeO2 content in lung tissue was significantly increased on day 1 only and the elevated level was decreased in a time-dependent manner to reach values found in control animals by day 14. These data suggested that a lung clearance of nano-CeO2 occurred over time. Nevertheless, the authors did not discuss possible contamination of the respiratory tract that could have occurred during gavage.
According to the authors, most of the administered nano-CeO2 was not absorbed into blood stream but excreted through faeces. In addition, the authors described no systemic toxicity in rats orally exposed to 100 and 5000 mg nano-CeO2/kg: there was no mortality, no change in serum biochemistry and haematology, and no histopathological lesion (see in section 7.2 Acute Toxicity). In conclusion, in this study again, nano-CeO2 displayed a low bioaccumulation and high faecal excretion.
Then, He X et al. (2010) employed the radiotracer technique to study the impact of nano-CeO2 uptake through the gastro-intestinal (GI) tract into rats exposed via oral route. There was no mention to any guideline or GLP. In addition, the statistical analyses were insufficiently documented and some methods were not clearly described which made the interpretation of the results difficult (e.g., no control animals). Therefore, the study was awarded a reliability score of 3 (Klimisch, 1997) and was flagged as supporting study.
Radiolabelled nano-CeO2 was used in this study. The physico-characterisation was performed on non-radioactive nano-CeO2, thus supposing that radioactive nano-CeO2 had the same physico-chemical properties as its non-radiolabelled counterpart. The physico-chemical characterisation is displayed in Table 1 above.
Three groups of male Wistar rats (6/group) were exposed once by gavage to 1 mL of a suspension of 1 mg/mL radiolabelled nano-CeO2 (corresponding to 4.7–5.3 mg/kg bw). Animals were then sacrificed at days 1, 3, and 7 post-exposure. Ce content, based on radioactivity measurements, was quantified in blood, lungs, heart, liver, kidney, spleen, stomach, intestine, testicle, brain, left femur, skeletal muscle, faeces, and urine.
In this gavage study, it was found that over 90% of the gavage dose was excreted during the first day and the administered dose was almost excreted completely (over 99%) within faeces during the first 3 days after administration. The highest contents of nano-CeO2 were found in stomach and intestine (up to 1 ng/g wet tissue at day 7 post-administration), the lowest in testicle and blood: blood (≤ 0.001 ng/g at day 7) < testicle < brain < lung (≤ 0.01 ng/g at day 7) < heart < spleen < kidney <muscle < bone (≤ 0.1 ng/g at day 7) < liver < intestine < stomach (≤ 1 ng/g at day 7) at days 1, 3, and 7. Nano-CeO2 was thus barely absorbed in the GI tract, though a large amount of nano-CeO2 was orally introduced. Moreover, the transit of particles through the GI tract did not seem to contribute to the increases of nano-CeO2 in the extrapulmonary organs.
Hirst SM et al. (2011) investigated the distribution of nano-CeO2 in orally-exposed mice. There was no mention to any guideline or GLP. In addition, the study displayed some restrictions regarding the level of details available on test animals (e.g., source, age, weight) and on the physico-chemical characteristics of the tested nano-CeO2; but, at the publication date, the scientific community required less physico-chemical endpoints in such articles. Nevertheless, toxicokinetic data on control animals were not provided in the publication. Thus, it was difficult to assess the biological relevance of CeO2 distribution in investigated tissues. Moreover, there was no data available regarding the experimental validation of the detection method, ICP-MS (e.g., limit of detection). Therefore, the publication was awarded a reliability score of 3 (Klimisch, 1997) and was flagged as supporting study.
In-house synthesised nano-CeO2 was used in this study. The physico-chemical characterisation is displayed in Table 1 above.
Female CD-1 mice (at least 3/group) were orally dosed once a week for 2 or 5 weeks with 0 (vehicle control: PBS) or 0.5 mg/kg nano-CeO2 as a suspension. Animals were sacrificed 1 week after the last administration. A histological examination of major organs (brain, lungs, liver, spleen, kidneys, and pancreas) was performed using light (LM) and electron microscopy (EM). Nano-CeO2 distribution was evaluated in organs using LM, EM, immuno-histochemistry (IHC) and ICP-MS. Moreover, immune responses were investigated by counting white blood cells.
The authors demonstrated that mice excreted through faeces around 98% of the administered nano-CeO2 within 24 h. This was probably due to a lack of uptake by the GI system. The remaining nano-CeO2 was mainly found in the lungs of orally exposed mice (ca. 1 ± 1 µg Ce/g at the end of 5-week exposure) and this was most likely due to slight aspiration according to the authors. There was no distribution of nano-CeO2 in the heart and brain of any mice. Moreover, nano-CeO2 did not cross the blood-brain barrier (BBB). In addition, nano-CeO2 showed no overt toxicity and no induction of immune responses.
In conclusion, here again, the tested nano-CeO2 displayed the classical behaviour of poorly soluble particles administered by oral route: low bioaccumulation and high faecal excretion.
Furthermore, Kumari M et al. (2014) investigated the biodistribution of nano-CeO2 in rats exposed repeatedly by oral route. This assay was part of a repeated-dose toxicity study performed according to the OECD guideline 407. However, there was no mention to GLP. The study was designed to compare nano-CeO2 to bulk CeO2 (or micro-CeO2), thus the latter was included in this study. Nano-CeO2 seemed insufficiently characterised for such recent publication (e.g., no data on surface properties). Furthermore, confidence in results of tissue distribution was reduced. Indeed, large Ce contents and really low standard deviations were observed in sometimes unexpected tissues/compartments from treated and control animals and that regardless of sexes, although the measurement method employed (ICP-optical emission spectrometry) is normally used to detect low contents in complex media but is known to display an inadequate sensitivity for some trace elements and a risk of element interferences in complex environments. In addition, animals used showed a really low body weight in comparison to standards of this strain at the same age. Therefore, the study was awarded a reliability score of 3 (Klimisch, 1997) and was flagged as disregarded study.
The physico-chemical characteristics of the commercial nano-CeO2 and bulk CeO2 used in this study are detailed in Table 1 above.
Male and female Wistar rats (5/sex and dose) were daily exposed to 0 (vehicle control: Milli-Q water), 30, 300 and 600 mg CeO2/kg bw)/day by gavage for 28 days. Body weight and food consumption were monitored weekly for 4 weeks. All rats were sacrificed by cervical dislocation 24 h after the last exposure to the vehicle or the test materials. Then, tissue samples were collected (i.e., urine, faeces, whole blood, liver, kidneys, heart, brain, and spleen). Ce content in the samples was determined using ICP-optical emission spectrometry (ICP-OES).
According to the authors, absorption and significant tissue distribution of nano-CeO2 and micro-CeO2 was observed in a dose-dependent manner. Kumari M et al. stated that there was a significant accumulation of Ce in all the tissues, i.e., liver, spleen, kidneys, heart, and brain in animals treated with nano-CeO2 through translocation into the blood. Furthermore, the intake of Ce in various tissues was very high in nano-CeO2 treated groups when compared to bulk CeO2-treated groups at all the dose level. The distribution of Ce was maximal in liver, kidneys, spleen, and blood from male and female rats treated with nano-CeO2 at the dose of 600 mg/kg bw/day followed by 300 and 30 mg/kg bw/day. Significant accumulation of Ce was observed in male and female rats treated with bulk CeO2 at 300 and 600 mg/kg bw/day in the liver and spleen, whereas in kidneys and blood only at 600 mg/kg bw/day. A small but significant amount of Ce in nano-CeO2 and bulk CeO2 treated rats was excreted in urine (3 to 9.5 µg/mL). Further, a large amount of particles was rapidly removed from the GI system into faeces (38 to 270 µg/g) for both test materials. It has to be noted that distinct difference in the data among genders, organs tested, forms (nano versus bulk) and the dose groups was not obvious. Using all Ce content values provided in the article, it was found that the calculated median value was around 3 whatever the experimental conditions including control animals. In addition, the authors described a systemic toxicity in rats orally exposed to 300 and 600 mg nano-CeO2/kg bw/day: clinical chemistry and histopathological findings, notably in the liver, and genetic damage, but no severe distress symptoms and mortality (see in sections 7.5 Repeated dose toxicity and 7.6 Genetic toxicity). Of note that high Ce contents were also detected in extrapulmonary organs and blood (1.7 to 3 µg/g), in urine (ca. 2.2 µg/mL) and faeces (< 10 µg/g) from control males and females. As seen for treated rats, distinct difference in the data among control genders was not obvious. These results are in contradiction with those described above by Hirst SM et al. or Molina RM et al. that did not evidence CeO2 in urine from mice weekly dosed with 0.5 mg nano-CeO2/kg for 2 or 5 weeks or in rats dosed once with 5 mg nano-CeO2/kg, both by oral route.
The authors concluded that prolonged oral exposure to high doses of nano-CeO2 had the potential to cause retention in vital organs of rats. However, the authors did not discuss possible contamination of tissues during sampling and/or analysis or methodology issues.
Discussion: oral route
Overall, published data on distribution and elimination of nano-CeO2 after oral exposure demonstrated that the physico-chemical characteristics of the tested nano-CeO2 seemed to have no or few impact on the nanoparticle toxicokinetic. Indeed, nearly the entire nano-CeO2 dose administered by oral route was systematically excreted through faeces (> 90-99%), while not more than 0.005% of nano-CeO2 dose was found in urine (He X et al., 2010; Hirst SM et al., 2011; Molina RM et al., 2014; Park EJ et al., 2009) whatever the primary particle size, the shape, the specific surface area or the oxidation state (see in Table 1 above). Extremely low absorption through GI tract and rare distribution to organs was described in animals orally exposed to nano-CeO2. He X et al. and Molina RM et al. reported a maximum content of nano-CeO2 in digestive tissues (i.e., around 1 or 2 ng/g in stomach and intestine); while Park EJ et al. and Hirst SM et al. described that the highest CeO2 deposition occurred in lungs (up to 35 µg/g tissue). However, as suggested by Hirst SM et al., the CeO2 contents found in lung tissues were most likely due to the gavage procedure or slight aspiration. This could explain the pulmonary accumulation of nano-CeO2 seen by Park EJ et al. and Hirst SM et al. and which was followed by a fast removal as described by Park EJ et al., although no or few administered nano-CeO2 was absorbed into blood stream. Indeed, using brightfield and enhanced darkfield microscopy and ICP-MS, no CeO2 nanoparticles were found in the lung and the liver of C57BL/6 female mice 1, 28 and 180 days after a single oral exposure to 162 µg nano CeO2 (primary particle size : 13.0 +/- 12.1 nm) (Modrzynska J et al., 2018).
There are however no data to evaluate the metabolization of nano-CeO2 by oral route. No conclusion can therefore be made regarding the transformation of these nanoparticles and/or its degradation products or metabolites by hepatic microsomal fractions.
In conclusion, nano-CeO2, when orally administered, seems to behave like its micrometric counterpart: i.e., as poorly soluble particles that are mainly and rapidly excreted through faeces with no or few absorption, distribution and/or metabolization. Moreover, the physico-chemical characteristics of nano-CeO2 appear to have no or few impact on the nanoparticle toxicokinetic when compared to its micrometric counterpart. This could be partially due to agglomeration and/or aggregation and insolubility of nano-CeO2 in physiological fluids; thus, in GI tract, the nanoparticles could behave as a micrometric CeO2 powder.
RESPIRATORY ROUTE (INHALATION AND INTRATRACHEAL INSTILLATION)
Five published studies are currently available on inhaled nano-CeO2 with various physico-chemical properties (Tentschert et al., 2020; Geraets L et al., 2012 (both by inhalation); He X et al., 2010 and Molina RM et al., 2014/Konduru etal., 2015 (by intratracheal instillation); these publications are thus used to conclude on the toxicokinetic behaviour of nano-CeO2. These in vivo studies were conducted in rats acutely or repeatedly exposed to the nanoparticles.
Inhalation
The CeO2 organ burden, distribution and localisation was determined in Wistar female ras by Tentschert J et al. (2020) as part a chronic whole-body inhalation study performed according to OECD TG 453 and in compliance with GLP. Groups of female rats were whole body exposed to nano CeO2 NM-212 (see the chararcteristics reported in the table 2 below, as reported in the article), 6 h per day for 5 days per week for 104 weeks with the following concentrations: 0 (control-air), 0.1, 0.3, 1.0, and 3.0 mg CeO2/m3. It should be noted that the physico-chemical characteristics reported below differed, at leat for the primary particle size, to those reported in the article of Keller (2015), while coming from the same study (carcinogenicity study, waiting for publication).
Table 2: Summary of the physico-chemical characteristics available on the nano-CeO2 tested by inhalation route
Physico-chemical parameters |
Tentschertet al. (2020) |
NM-212 |
|
Supplier |
No data * |
Synthesis method |
No data |
Primary particle size |
28.4 nm |
Particle size distribution -MMAD |
1.4 to 2.3 µm |
Stability |
Agglomeration / aggregation |
Specific surface area |
27.2 m²/g |
Surface charge |
No data |
Isoelectric point |
No data |
Shape |
No data |
Crystallinity |
No data |
Purity / impurities |
> 99.5 % |
Solubility |
< 1 µg/L |
Oxidation degree |
No data |
Surface properties |
No data |
Number concentration of particles in aerosol |
No data |
*The NP characterization was done by the European Commission Joint Research Center (JRC) Nanomaterial Repository (Ispra, Italy).Information provided by Singh et al. (2014) and Fh-IME Schmallenberg.
The CeO2 burden was measured in lung, lung-associated lymph nodes, brain, olfactory bulbs, kidneys, liver, heart, spleen, small intestine (jejunum), bone (femur), bone marrow, blood and in feces in 3 to 4 animals/groups after 3, 12, 24 months of exposure. Quantification of CeO2 organ burden and visualization of nanoparticule distribution in tissues were performed using ICP-MS and ion-beam microscopy. Experimentally quantified lung burdens were compared with predictions of the deposited alveolar burden using the software an MPPD model.
The lung and the lung associated lumph nodes (LALNs) were found to contain the highest burden of CeO2. After 24 months of exposure, the mean CeO2 lung burden was found to be 1 ± 0,3, 78 ± 35, 348 ± 54, 1450 ± 275 and 4412 ± 1030 µg/lung in groups exposed to 0, 0.1, 0.3, 1.0, and 3.0 mg/m3, respectively. For all aerosol concentrations tested, a linear increase in lung burden was observed over time. The alveolar deposition fraction was estimated using MMPD model around 12% for all exposed groups. The nanoCeO2 contents of the lung associated lymph nodes reach of level of 2372 ± 560 µg CeO2/organ in the high dose concentration group after 24 months of exposure whereas the CeO2 content in mesenteric lymph nodes in the same animal group was = 73 +/- 20 ng/organ. Inhomogenous nanoparticle agglomerates were foung throughout the whole lung. Large NP agglomerates (several up to > 200 mm²) are concentrated in the bronchus-associated lymphoid tissue (BALT) with smaller agglomerates distributed throughout the remaining lung tissue. The particles were found mainly in macrophages. In addition, the alveolar septum contained some smaller NP agglomerates.
The content of CeO2 in all extrapulmonary organ was very low according to the authors. Besides the lung associated lymph nodes, CeO2 burden was highest in liver (12 +/- 3 µg/organ) and relatively high amounts of Ce was found also in femur bones (~1.8 +/- 0.4 µg/g) and bone marrow (~0.350 +/- 0.1 µg/g) in animals of group 4 after 24 month of exposure in comparison to all other extrapulmonary organs, except LALNs. The burden of the skeleton (calculated from CeO2 concentration in femur tissue) in the same animal group was calculated to be 44.9 µg/skeleton. CeO2 burden in other organs were negligible. The following ranking was proposed by the authors lungs > lymph node > hard bone > liver > bone marrow > kidneys, spleen >>> heart > brain > olfactory bulb. According to the authors, under these assumptions, around 1.1% of the lung burden would be retained in the skeleton for the group 4. In comparison, 1.2% of the lung burden was found in all investigated extra-pulmonary organs excluding lymph nodes and skeleton.
According to the accumulation rates, the authors concluded that, with a low accumulation rate, the liver can be regarded as a depot, whereas kidneys, the skeleton and bone marrow seem to be dumps due to steadily increasing nanoparticle burden over time.
It should be noted that in all examined extrapulmonary organs and histopathological examinations of the rats of this study, there was no detection of any adverse effects and no indications of systemic toxicity were found in any of the examined organs (see Keller et al, 2015 and Schaudien et al, 2019, in IUCLID section 7.5.2).
Geraets L et al. (2012) investigated blood and tissue kinetics of nano-CeO2 and micro-CeO2 during and after a 28-day inhalation study in rats. There was no mention to GLP. Though performed according to OECD guidelines, the study displayed some restrictions. First, there was no comparison with controls animals while background Ce levels in these animals were observed by other authors. Moreover, there were only 3 animals per group and a high variability (as standard deviation) for almost all results. In addition, results were expressed as µg Ce/kg organ weights but without any precision on whether wet or dry tissue was concerned. Furthermore, organ weights were not measured in the study, data came from another study. There were imprecisions regarding the total inhaled dose (i.e., not clear if data reported in the article related to single or repeated exposure), no clear data regarding detection limit(s) from the apparatus used (ICP-MS and ICP-AES) in the study. The design of repeated exposures was not equivalent in term of number of exposure for each animal. At last, the interpretation of data could be confusing as the way results were expressed changed for each figure. The study was awarded a reliability score of 2 (Klimisch, 1997) and was flagged as supporting study.
The study was performed using two nano-CeO2 (Antaria NM-211; Umicore NM-212) and one micro-CeO2 (Sigma-Aldrich NM-213); powder aerosolization resulted in comparable mass median aerodynamic diameter (MMAD): 1.17 µm for NM-212, 1.02 µm for NM-211 and 1.40 µm for NM-213, meaning that particles aggregated in the test atmosphere. The physico-chemical characteristics of nano- and micro-CeO2 are detailed in Table 2' below.
Table 2': Summary of the physico-chemical characteristics available on the various nano-CeO2 tested by inhalation route
Parameters |
Geraets L et al. (2012) |
|
|
|
NM-211 |
NM-212 |
NM-213 |
Supplier |
Antaria |
Umicore |
Sigma-Aldrich |
Synthesis method |
No data |
No data |
No data |
Primary particle size |
13.0 nm |
27.3 nm |
> 500 nm |
Particle size distribution (mass media, aerodynamic diamter) |
1.02 µm |
1.17 µm |
1.40 µm |
(mass median aerodynamic diameter) |
1.02 µm |
1.17 µm |
1.40 µm |
Stability |
Agglomeration / aggregation |
Agglomeration / aggregation |
Agglomeration / aggregation |
Specific surface area |
63.65 m²/g |
27.15 m²/g |
3.73 m²/g |
Surface charge (zeta potential) |
No data |
No data |
No data |
Isoelectric point |
No data |
No data |
No data |
Shape |
No data |
Polydispersed |
No data |
Crystallinity |
No data |
No data |
No data |
Purity / impurities |
99.5% purity |
99.95% purity |
99.5% purity |
Solubility |
Extremely poor in water at neutral pH |
Extremely poor in water at neutral pH |
Extremely poor in water at neutral pH |
Oxidation degree |
No data |
No data |
No data |
Surface properties |
No data |
No data |
No data |
Number concentration of particles in aerosol |
1.79 x 10E6 particles/cm3 |
1.1 x 10E6 particles/cm3 |
0.68 x 10E6 particles/cm3 |
Male Wistar rats (3/group) were exposed to CeO2 via inhalation route (nose-only), 6 h/day for 1 to 28 days with a number of exposures ranging from 1 to 20. Moreover, recovery groups were included in this study which consisted in sacrifice of rats 48 or 72 h instead of within 1 h after the last exposure. Though mentioned in the publication, there was no clear description on control animals. Particle concentrations were targeted to be the same: 1.79 x 10E6 particles/cm3 for NM-211, 1.1 x 10E6 particles/cm3 for NM-212, 0.68 x 10E6 particles/cm3 for NM-213. However, the determination of CeO2 mass concentrations by thermogravimetric analysis demonstrated that CeO2 concentrations were different: 10.79 mg/m3 for NM-211, 19.95 mg/m3 for NM-212, 55.00 mg/m3 for NM-213.
Geraets L et al. observed that CeO2 concentration in blood after single and repeated exposure was below the detection limit (e.g., < 5 µg/kg) for most samples. The authors suggested that this was due to a fast distribution of CeO2 from blood to tissues.
After single exposure, approximately 5 to 10% of the inhaled dose was measured in lung tissue, as was also estimated by a multiple path particle dosimetry model (MPPD). Though small differences in pulmonary deposition efficiencies of CeO2 were observed, no consistent differences in pulmonary deposition between NM-211, NM-212, and NM-213 were observed. After a single 6-h exposure, each CeO2 sample was also distributed to extrapulmonary tissues with an order of concentration of spleen (< 0.006%) ≤ epididymis (< 0.015%) ≤ kidney (< 0.015%) ≤ testicles (< 0.02%) ≤ brain (< 0.04%) < liver (< 0.15%, as maximal values as percent of inhaled dose). No clear particle size- or surface area-dependent effect on pulmonary deposition and extrapulmonary tissue distribution was observed.
According to the authors, repeated exposure to CeO2 particles resulted in significant accumulation of the particles in lungs and extrapulmonary tissues.
In addition, tissue clearance was shown to be slow, and, overall, insignificant amounts of CeO2 were eliminated from the body within the 48- to 72-h post-exposure period. However, CeO2 elimination was estimated based only on CeO2 contents in the investigated tissues; CeO2 quantification in faeces or urine was not included. Moreover, it seemed difficult to draw any conclusion on CeO2 elimination given the very low but highly variable levels of CeO2 found in extrapulmonary organs and given the lack of data on control animals.
The authors suggested that the lack of correlation between primary particle size and pulmonary deposition or extrapulmonary distribution was likely due to the presence of aggregates rather than single nano-sized particles in the test atmosphere resulting in similar aerodynamic diameter of micro- and nano-CeO2 but with different surface characteristics. The authors mentioned that these findings did not support the hypothesis that specific surface area is a good predictor of either the pulmonary or extrapulmonary dose for adverse effects.
Modelisation of lung deposition using MPPD displayed results in agreement with the data obtained in the experimental study: 7, 8, and 9% of inhaled NM-213, -212, -211, respectively, were expected to be deposited in the lungs. Based on deposition modelisation, the olfactory deposition of the tested CeO2 was expected to be minimal, even though the deposition in the head region was relatively high. Thus, the olfactory route did not seem to have contributed to the contents of CeO2 observed in the brain. Contrarily, this content could have resulted from contamination during autopsy.
In addition, according to Geraets L et al., no differences among both nano-CeO2 and micro-CeO2 were seen in term of pathology or other markers of toxicity.
In conclusion, following single and repeated inhalation, the tested nano-CeO2 and micro-CeO2 were deposited and mainly accumulated in the lungs, irrespective of the primary particle size of each CeO2 tested. No clear effect of the primary particle size or surface area on pulmonary deposition and extrapulmonary tissue distribution was observed, probably due to presence of aggregates. The data available suggested a possible accumulation in extrapulmonary tissues but CeO2 contents in the investigated tissues were extremely low. Geraets L et al. appropriately mentioned that they could not rule out that a contamination of extrapulmonary tissues had occurred during autopsy, thus explaining the low but detectable amounts of CeO2 found in these organs. Furthermore, insignificant amounts of CeO2 were eliminated in 48-72h.
Intratracheal instillation
Three other published studies are available and both used a non-physiological route of administration: the intratracheal (i.t.) instillation (He X et al., 2010; Molina RM et al., 2014/Konduru et al. 2015).
As presented above (for oral route), Molina RM et al. (2014)/ Konduru et al., investigated the bioavailability, tissue distribution, clearance and excretion of a neutron-activated 141nano-CeO2 after i.t. instillation to rats. There was no mention to any guideline or GLP. Nevertheless, the studies were well documented and met the generally accepted scientific principles. Thus, it was awarded a reliability score of 2 (Klimisch, 1997) and was flagged as weight of evidence.
The commercial nano-CeO2 (NM-212) powder or in the in-house synthesized nano-CeO2 were neutron activated; the process generated the radioisotope 141Ce. The physico-characterisation was performed on non-radioactive nanoparticles, thus supposing that radioactive nano-CeO2 had the same physico-chemical properties as its non-radiolabelled counterpart (see in Table 3 below).
Table 3: Summary of the physico-chemical characteristics available on the various nano-CeO2 tested by intratracheal route
Parameters |
He X et al. (2010) |
Molina RM et al. (2012) / Konduru et al., (2015) |
|
- |
NM-212 / In-house synthesized |
Supplier |
None (in-house synth.) |
Mercator GmbH / - |
Synthesis method |
Precipitation method |
No data / Flame spray pyrolysis |
Primary particle size |
6.6 ± 0.9 nm |
40 nm / 32.9 nm |
Particle size distribution |
12.8 ± 2.2 nm in aqueous solution |
207 to 432 nm in distilled water / 136 +/- 1 nm |
Stability |
Agglomeration / aggregation |
Agglomeration (35 nm to 7 µm) / agglomeration |
Specific surface area |
86.85 m²/g |
27 m²/g / 28 m²/g |
Surface charge (zeta potential) |
+30.1 mV |
+37 to +42 mV in water / +34.5 +/- 3.1 mV |
Isoelectric point |
No data |
> pH 10 (always cationic) in water / - |
Shape |
No data |
Roughly globular / - |
Crystallinity |
Crystalline (cubic, fluorite) |
Crystalline (cubic, cerianite) / - |
Purity / impurities |
No data |
ca. 99.3% purity / - 0.7% organic contaminations (ester + alkyl groups) on the particle surface as a very thin and homogeneous layer around the pure inorganic particles / - |
Solubility |
Insoluble (ca. 0.12%) at neutral and acid pH |
Insoluble in various media / - |
Oxidation degree |
No data |
Mainly as Ce(IV) but 14% as Ce(III) / - |
Surface properties |
No data |
Surface chemistry composed of 79.9% C, 17.7% O and 2.4% Ce / - |
- No data
in both studies, male Wistar rats (at least 5/group) were exposed once to 0 (vehicle control: distilled water) or 1 mg/kg of nano-CeO2 by i.t. instillation. All treated rats were sacrificed 5 min, 2, 7 and 28 days post-administration. Then, the following tissue samples were collected: blood, plasma, serum, lung, bone marrow, femoral bone, skin, brain, skeletal muscle, testicles, kidneys, spleen, heart, liver, stomach, small intestine, large intestine, and caecum. Moreover, 24-h samples of faeces and urine were collected at selected time points (0–24 hours, 2–3 days, 6–7 days, 9–10 days, 13–14 days, 20–21 days and 27–28 days post-gavage). The Ce content in the samples was determined by measuring the radioactivity (141Ce) using a gamma counter. BALF parameters such as macrophages, lymphocytes, PMN or LDH were first measured in order to evaluate whether the concentration used for the instillation would induce lung injury.
The selected i.t. instillation dose of 1 mg/kg nano-CeO2 did not cause major lung injury and inflammation. Sequential analyses of lungs over 28 days showed a high accumulation in the lungs (81-88.3% of the instilled dose after 28 days) and slow lung clearance of 141nano-CeO2 (estimated half-life of ca. 140 days). Translocation of nano-CeO2 to extrapulmonary organs was very low in both studies. About 0.86% to < 1% of instilled 141Ce was measured in selected extrapulmonary organs from 141nano-CeO2 groups, notably in liver (0.26% of the instilled dose) and bone (0.32% of the instilled dose). The elimination of 141Ce from 141nano-CeO2 occurred mostly through faeces (~ 8.5 to 19 % of the instilled dose) and to a much lesser extent through urine (~ 0.015 to 0.048 % of the instilled dose).
According to the authors, the slow clearance of nano-CeO2 might be due to poor solubility of CeO2, as shown by the absence of dissolution in simulant phagolysosomal fluid. Moreover, the significant association of nano-CeO2 with alveolar cells and lung interstitial tissues may have promoted its biopersistence.
Molina RM et al. concluded that, based on these results, low fractions of inhaled nano-CeO2 were expected to translocate to extrapulmonary tissues. Furthermore, the risk of potential toxicity was expected to be low in case of nano-CeO2 inhalation. Further, the authors said that the lung clearance observed in both studies that used 2 different nanoCeO2 were similar.
He X et al. (2010) also employed a radiotracer technique to study the pulmonary deposition and the translocation of radiolabelled nano-CeO2 to secondary target organs in i.t. instilled rats. There was no mention to any guideline or GLP. Moreover, the statistical analyses were insufficiently documented and some methods and results were not clearly described, which made it difficult to understand (e.g., no controls animals). Therefore, the study was awarded a reliability score of 3 (Klimisch, 1997) and was flagged as supporting study.
Radiolabelled nano-CeO2 was used in this study. The nanoparticles were synthesised from radioactive Ce(NO3)3 using a precipitation method. However, as the authors assumed that the radiolabelled and non-labelled nanoparticles had the same physico-chemical properties, only the non-radiolabelled nano-CeO2 was characterised (see in Table 3 above).
Male Wistar rats (6/group) were i.t. instilled with 0.1 mL of a nano-CeO2 suspension at 2 mg/mL in Milli-Q ultrapure water (sonicated for 15 min before use), and then sacrificed at 6 h, 24 h, 7 d, and 28 d after exposure. Ce content, based on radioactivity measurements of 141Ce, was quantified in blood, lungs, heart, liver, kidney, spleen, stomach, intestine, testicle, brain, left femur, skeletal muscle, faeces, and urine using a photon detector.
The study showed that 24 h after exposure nearly 80% of the instilled nano-CeO2 was deposited in the lung regions and 63.9% of the particles remained in the lung by 28 days post-exposure. The deposited nano-CeO2 was cleared slowly from the host lung. The initial clearance rate was calculated to be 1.03 µg/d and the elimination half-life was 103 days. Nano-CeO2 content in blood slightly increased within 10 min after instillation (< 10E-6 % from 6 h to 28 days post-i.t.) and the transported particles could be found in extrapulmonary tissues suggesting that intratracheally instilled nano-CeO2 could penetrate through the alveolar-capillary barrier into the systemic circulation and accumulate in the following extrapulmonary organs: liver (0.0005% and 0.1% at 6 h and 28 days post-i.t., respectively), spleen (≤ 0.0001% and 0.01% at 6 h and 28 days post-i.t., respectively), and kidney (0.00005% and ≤ 0.005% at 6 h and 28 days post-i.t., respectively). Though the particle fractions in liver and spleen demonstrated a tendency towards time-dependent increase and that nano-CeO2 contents increased by two orders in both organs during the test period, such a trend was not apparent in other organs like stomach or heart. Nano-CeO2 contents remained at a low level in other tissues from 6 h to 28 d post-i.t. (< 10E-6 % to ≤ 0.001% for brain, testicles, heart, or stomach). At the end of the test period, only 1/8-1/3 of the daily elimination of nano-CeO2 from the lung was cleared via the GI tract, suggesting that phagocytosis by alveolar macrophages (AMs) with subsequent removal towards the larynx was no longer the predominant route for the elimination of nano-CeO2 from the lung. According to He X et al., most of the nano-CeO2 lost from the lungs could be found in faeces and little could be detected in urine samples (below 1 x 10E-7 µg/g at each time point). However, during the first 3 days, the nano-CeO2 content in faeces decreased sharply. He X et al. suggested that the major parts of excreted nano-CeO2 might be those directly returned from the lungs to the larynx or removed via mucociliary clearance.
Even though the results described in this publication seemed in adequacy with previous studies on nano-CeO2 biodistribution, it was difficult to assess the relevance of these data given the lack of statistics, some unclear descriptions of the methods, and the absence of control animals. The latter point would have been interesting since some data on Ce content in blood or tissues were so low that this could either reflect a background level of Ce or the result of a contamination.
As seen in the study of Molina RM et al. (2014), He X et al. (2010) using intratracheal instillation detected a higher accumulation of nano-CeO2 in the lungs (~80%) than in the inhalation study from Geraets L et al. (< 13%).
Discussion: inhalation - intratracheal route
All 5 published studies demonstrated, overall, that nano-CeO2 administered by respiratory route (inhalation or i.t.) was mainly accumulated and retained in lungs and lung asociated lymph nodes, weakly absorbed from lungs to the blood, quickly but poorly distributed from the blood to extrapulmonary tissues, and very poorly eliminated from tissues. From the studies that quantified nano CeO2 in extrapulmonary organs, around 0,86 to 1,2 % was found to be distrbuted in those extrapulmonary organs excluding the skeleton, in which Tenschert J. et al. (2020) and Molina RM et al. (2014) found the highest content of nano CeO2 (estimated ~1,1 %, and 0.32 %, respectively). Then, the order of the extrapumony organs containg nanoCeO2 may differ in the 5 studies analysed, probably due to the experimental procedures implemented. However, the liver appear in all these studies as the next organ involved in the clearance of nano CeO2 (0,26 to 0,1 %) followed, in a lesser extent by spleen (0,1 to 0,006 %) and/or kidneys (0,015 to 0,005 %) and low to negligible CeO2 content were found in the others organs studies (such as heart, brain, olfactory bulb or testes). The clearance rate from lungs was estimated to be low with an elimination half-life ranging from 103 to 140 days in i.t. studies. However, the lung accumulation of nano-CeO2 was higher in i.t. studies (63 to 88%) than in the inhalation studies (< 5 to 12%). This difference might be explained by the route of administration employed by He X et al. (2010) and Molina RM et al. (2014)/Konduru et al. (2015). Indeed, the i.t. instillation might have allowed nano-CeO2 to reach the deep lungs as a single and massive bolus, thus artificially increasing the accumulation rate of CeO2 particles in the pulmonary compartment. It has to be noted that nanomaterials such as nano-CeO2 entering the lung and destined for the blood stream would need to cross at least 10 separate biological barriers before reaching their target (Walkey C et al., 2015). The physico-chemical characteristics such as primary particle size, the aging process of the particles by interaction with other ambiant airbone pollutants or specific surface area of the tested CeO2 (nano and bulk) seemed to have no or few impact on the particle toxicokinetic. Indeed, Li D et al. (2016) who analysed the bioditribution of fresh and aged nano ceria in SD male rats after an acute inhalation exposure found no difference in particles biodistribution between fresh and aged CeO2 NP and more particularly when the smaller particles (< 70 nm) were considered. Further, Arts JHE et al. (2015) noted that cumulative lung burden depends on the size of agglomerated particles and the likelihood of their disintegration. Nanomaterial agglomerates that form in aerosols and do not disintegrate in the lung are unlikely to become systemically available. In the studies of Geraets L et al. (2012) and Tentschert J et al. (2020) , the tested nano-CeO2 agglomerated and reached sizes from ca. 1 µm up to to 7 µm. These large agglomerate sizes might, at least partially, explained the poor extrapulmonary distribution described in these studies. The fraction of CeO2 that was eliminated was mainly found in faeces and really few were detected in urine.
Further, Tentschert J et al. (2020), using imaging mass spectrometry, identified CeO+ ions and cerium phosphate ions in the femur sections. According to the authors, this indicates particle dissolution of CeO2 and the formation of cerium-ions on the way from lung to bone or the formation of cerium ions from particles directly in the bone.
In conclusion, nano-CeO2, when administered by respiratory route, seemed to behave like poorly soluble particles that are mainly retained in the lungs and are only poorly absorbed, distributed (to extrapulmonary tissues such as liver, spleen and skeleton), cleared from tissues and/or eliminated (mainly through faeces via GI tract). Regarding its metabolization, nano-CeO2 as an inorganic compound is not expected to undergo significant metabolism but a transformation instead may occur. Indeed, Tentschert J et al. (2020) have observed cerium phosphates in bone that could result from cerium oxide dissolution and transformation to cerium phosphate in this tissue. Further, an hepatic biotransformation of i.v.-injected nano-CeO2, consisting in a partial dissolution causing a shift towards smaller particle size with a measurable change in the valence reduction (38 to 70% Ce(III)) and redox activity, has been observed by Graham UM et al. (2014) and Modrzynska J et al. (2016) (see below in chapter "Other routes") after prolonged residence time in the liver (90 -180 days). The physico-chemical characteristics of nano-CeO2 appeared to have no or few impact on the nanoparticle toxicokinetic when compared to its micrometric counterpart. This could be partially due to agglomeration and/or aggregation and insolubility of nano-CeO2 in physiological fluids.
DERMAL ROUTE
Regarding the dermal route, one in vitro study testing nano-CeO2 absorption in intact and damage human skin is available (Mauro M et al., 2019). The study was performed according to OECD guideline n° 428, but no information on the GLP status was indicated in the article. Thus, the study was scored as validity 2 according to Klimisch criteria.
The experiments were performed using intact and needle-abraded human abdominal full-thickness skin obtained from 2 females donors. Percutaneous absorption of nanoCeO2 was studied using static diffusion cells. The exposure chambers of three Franz dffusion cells were filled with 0.6 mg/cm2 (corresponfing to 1g/L) of the in-house synthesized CeO2 (size distribution: 17 nm) dispersed in synthetic sweat. After 24 h, the receiving solutions and skins sample were removed and quantitative analyses of nano CeO2 were done.
The average amounts of Ce into intact and damaged whole skin samples measured at the end of the 24-hour exposure period were 3.64 +/- 0.15 and 7.07 +/- 0.78 µg/cm2, respectively. Ce concentration in the receiving solution was 2.0 +/-0.4 (as in the blank cells) and 3.3 +/- 0.7 ng/cm2 after 24 h. The Ce content was higher in dermal layers of damaged skin compared to intact skin (2.93 +/- 0.71 µg/cm2 and 0.39 +/- 0.16 µg/cm2, respectively).
The authors concluded that CeO2 NPs could not permeate intact skin, but this permeation was possible—if at a low level—using an abraded skin protocol. A low amount of cerium was present in intact and damaged skin samples after prolonged exposure (24 h) to CeO2NPs. They further suggested that this behavior is probably due to the very low ionization of these metal oxides in synthetic sweat, resulting in a small concentration of free metal ions in the donor phase being able to cross over the physiological barriers.
According to the results of this study, a systemic distribution of nano-CeO2 is not expected from dermal exposures. In addiition, given its poor absorption and its inorganic nature, these insoluble nanoparticles are considered to have weak or no potential for metabolization following dermal exposure.
In conclusion, when dermally applied, nano-CeO2 is expected to be poorly absorbed, distributed and/or metabolized.
OTHER ROUTES (INTRAVENOUS, INTRAPERITONEAL AND INTRAVITREAL EXPOSURE)
Additional 16 in vivo studies were available on various nano-CeO2 administered by i.v., intraperitoneal (i.p.) or intravitreal routes. Although these routes are not an expected route of exposure, and the nanoCeO2 used in these studies have in most case a modified surface (e.g. citrate coated), these studies were reported here because the results could provide information helping in understanding the behavior of cerium oxide once in the body. These studies were conducted in rats or mice by acute or repeated exposures to the nanoparticles.
Intravenous injection
There were 15 publications available on nano-CeO2 toxicokinetic behaviour in rodents exposed by i.v. injection (Dan M et al., 2012a and 2012b; Graham UM et al., 2014; Hardas SS et al., 2010, 2012 and 2014; Hirst SM et al., 2009 and 2011; Molina RM et al., 2014/Konduru et al., 2015; Rojas S et al., 2012; Yokel RA et al., 2009, 2012, 2013 and 2014).
Yokel’s research team published 10 articles, in which nano-CeO2 absorption, distribution and biotransformation were described in i.v. exposed rats (Dan M et al., 2012a and 2012b; Graham UM et al., 2014; Hardas SS et al., 2010, 2012 and 2014; Yokel RA et al., 2009, 2012, 2013 and 2014). Well documented and meeting generally accepted scientific principles, the studies were awarded a reliability score of 2 (Klimisch, 1997) and flagged as supporting study or weight of evidence.
Yokel RA et al. (2009) characterised the biodistribution of nano-CeO2 from blood after i.v. infusion and the effects on oxidative stress endpoints in vivo in rats. There was no mention to any guideline or GLP. Although the details provided on test animals seemed rather insufficient (e.g., no data on age, diet, acclimation period, or environmental conditions), the study was considered to meet generally accepted scientific principles. Therefore, the study was flagged as weight of evidence.
A water suspension (5%) of crystalline nano-CeO2 was used in this study. The physico-chemical characteristics of the tested nano-CeO2 are displayed in Table 4 below.
Table 4: Summary of the physico-chemical characteristics available on the various nano-CeO2 tested by intravenous route
Parameters |
Graham UM et al. (2014) |
Hardas SS et al. (2010, 2012) Dan M et al. (2012b) |
Hirst SM et al. (2009, 2011) |
Molina RM et al. (2012) / Konduru et al. (2015) |
|
- |
- |
- |
NM-212 / In-house synthesized |
Supplier |
None (in-house synth.) |
None (in-house synth.) |
None (in-house synth.) |
Mercator GmbH / - |
Synthesis method |
Hydrothermal method |
Hydrothermal method |
Wet chemistry method |
No data / Flame spray pyrolysis |
Primary particle size |
ca. 30 nm |
4.6 ± 0.1 to ca. 5 nm |
3 to 5 nm |
40 nm / 32.9 nm |
Particle size distribution |
31 ± 4 nm |
Bimodal distribution with peaks at 6.4 and 12.6 nm in water and > 70% of non-agglomerated particles and 98% of particles in the range of 6.7-8.2 nm |
10 to 50 nm |
207 to 432 nm in distilled water / 136 +/- 1 nm |
Stability |
No agglomeration / aggregation in aqueous suspension (due to citrate coating) |
(i) No agglomeration / aggregation in water at pH 7.7-8 and in perfusate at 37°C (ii) Small agglomeration in freshly rat blood |
Agglomeration |
Agglomeration (35 nm to 7 µm) / agglomerattion |
Specific surface area |
No data |
121 m²/g |
No data |
27 m²/g / 28 m²/g |
Surface charge (zeta potential) |
No data |
-53 mV in water at pH ~7.35 |
No data |
+37 to +42 mV in water / +34.5 +/- 3.1 mV |
Isoelectric point |
No data |
No data |
No data |
> pH 10 (always cationic) in water / - |
Shape |
Cubic |
Polyhedral |
No data |
Roughly globular / - |
Crystallinity |
Crystalline |
Crystalline (face-centred cubic) |
Crystalline (fluorite) |
Crystalline (cubic, cerianite) / - |
Purity / impurities |
No data |
Impurities: La traces (< 10E-5 wt%) |
No data |
ca. 99.3% purity / - 0.7% organic contaminations (ester + alkyl groups) on the particle surface as a very thin and homogeneous layer around the pure inorganic particles / - |
Solubility |
No data |
No data |
No data |
Insoluble in various media / - |
Oxidation degree |
No data |
High Ce(III) valence |
Mixing of Ce(III) and Ce(IV), with a relatively higher abundance of Ce(III) |
Mainly as Ce(IV) but 14% as Ce(III) / - |
Surface properties |
Citrate coating of surface |
40% of surface coated with citrate |
No data |
Surface chemistry composed of 79.9% C, 17.7% O and 2.4% Ce / - |
pH of nano-CeO2 suspension |
3.9 |
No data |
≤ 3.5 |
7.85 / - |
Parameters |
Rojas S et al. (2012) |
Yokel RA et al. (2009) |
Yokel RA et al. (2012) Hardas SS et al. (2014) |
|
Supplier |
None (in-house synth.) |
Sigma-Aldrich |
None (in-house synth.) |
|
Synthesis method |
Wet chemistry method |
No data |
Hydrothermal method |
|
Primary particle size |
5 nm |
31 ± 4 nm |
31.2 ± 17.1 nm |
|
Particle size distribution |
No data |
Bimodal distribution with peaks at 8 and 24 nm |
Bimodal distribution in water with peaks at 41 and 273 nm |
|
Stability |
No data |
Agglomeration |
Agglomeration / aggregation |
|
Specific surface area |
180 m²/g |
(0.2 to 1 µm), in freshly rat blood for 1 h |
15 m²/g |
|
Surface charge (zeta potential) |
+18.5 mV for functionalised but non-radiolabelled nano-CeO2 (in water, at neutral pH) |
No data |
-56 mV in water at pH ~7.3 |
|
Isoelectric point |
No data |
-7.6 mV at its original pH 4.2 and -35 mV at physiological pH 7.4 |
No data |
|
Shape |
No data |
No data |
Cubic |
|
Crystallinity |
Crystalline, with an average crystallite size of 5.6 nm |
Platelets |
Crystalline (face-centred cubic) |
|
Purity / impurities |
No data |
No data Impurities: Pb, Zn, Al, Fe, Cu, Ti, Ni (representing < 0.2%) |
99.1% purity |
|
Solubility |
No data |
No data |
No data |
|
Oxidation degree |
No data |
No data |
Enrichment of Ce(III) at the particle rim (surface layer) |
|
Surface properties |
No data |
Presence of organic molecules at the surface, probably either an intentionally added stabilizer or an unintentional contaminant |
ca. 18% of surface coated with citrate |
|
pH of nano-CeO2 suspension |
No data |
4.2 |
3.9 |
|
- No data
The nano-CeO2 suspension was i.v. infused once at 0 (vehicle control: water), 50, 250, 750 mg/kg into male Fisher rats (4 to 10/group), which were terminated 1 or 20 h later. Biodistribution in rat tissues was assessed by microscopy and ICP-atomic emission/ -mass spectroscopy (ICP-AES/MS). Oxidative stress effects were assessed by protein carbonyls (PC), 3-nitrotyrosine (3NT), and protein-bound 4-hydroxy-2 -transnonenal (4-HNE). Evans blue (EB)-albumin and Na fluorescein (Na2F) were given i.v. as BBB integrity markers.
Although some clinical signs of tachypnea, dyspnea or bruxism were observed in some rats, notably at the highest dose, no adverse effects were seen after completion of i.v. infusion. Moreover, no animal died prior to their planned termination. Nano-CeO2 was rapidly cleared from the blood to peripheral reticulo-endothelial tissues (t1/2 = 7.5 min). Much less CeO2 entered the BBB cells or the brain. Indeed, microscopy revealed peripheral organ (spleen, liver and kidney) CeO2 agglomerations but little in the brain. Spleen CeO2 concentration (up to ca. 32 g/kg wet weight 20 h post-i.v.) was higher than in liver (up to ca. 9 g/kg) > blood (up to 354 mg/L) > brain (up to 7.4 mg/kg). 4-HNE showed a trend toward increased levels in the hippocampus at 20h at dose levels ≥ 250 nano-CeO2 mg/kg. PC and 3-NT changes were small. However, in the cerebellum, only PC levels were decreased at 20h at dose levels ≥ 250 nano-CeO2 mg/kg, while the other oxidative stress markers were not changed.
In conclusion, given the large doses i.v. administered to rats, the commercial CeO2 tested showed limited toxicological effects notably in the brain. The authors concluded that this was consistent with the understanding of CeO2 as an inert material and that the lack of profound effects on the oxidative stress endpoints in the brain might be related to the limited CeO2 distribution into the brain. Thus, the BBB seemed to be an effective barrier for this crystalline nano-CeO2.
In another study, Yokel RA et al. (2012) ascertained the distribution, translocation, elimination and selected biological effects (including histopathology and oxidative stress) in rats after a single i.v. administration of nano-CeO2. In a second study from the same research team, Hardas SS et al. (2014) aimed at characterising the biodistribution of the same nano-CeO2 from blood into brain of additional rats up to 90 days after systemic administration. There was no mention to any guideline or GLP. However, the studies were well documented and met generally accepted scientific principles. Thus, both studies were summarised in the same Endpoint Study Record and were flagged as weight of evidence.
Both studies used the same in-house synthesised citrate-coated nano-CeO2 (at 5% in water suspension) that was extensively characterised (see in Table 4 above).
Male SD rats (3 to 11/group) were i.v. infused with water (vehicle control) or with a nano-CeO2 suspension at an analytical dose of 87 mg/kg. Rats were then terminated 1 and 20 hours, and 1, 7, 30, or 90 days post-exposure. Cage-side observations were performed daily and body weight weekly. Daily urinary and faecal Ce outputs were quantified for 2 weeks. Nine organs were weighed and samples collected from 14 tissues/organs/systems, blood and cerebrospinal fluid for Ce determination. A histological analysis was performed using EM. CeO2 concentration and chemical speciation in the brain were assessed by ICP-MS and electron energy loss spectroscopy (EELS). Oxidative stress was assessed in liver and spleen by measuring PC levels.
As previously observed by Yokel RA et al., no mortality and no adverse effects were seen after administration of 87 mg nano-CeO2/kg to rats.
The authors demonstrated that less than 1% of the administered nano-CeO2 was excreted in the first 2 weeks, with 98% of eliminated CeO2 found in faeces. Body weight gain was initially impaired. Spleen weight was significantly increased in some CeO2-treated groups, associated with abnormalities (e.g., punctate white specs). CeO2 was primarily retained in the spleen, liver (both organs of the mononuclear phagocyte system (MPS)), and bone marrow. There was no relevant decrease of CeO2 in any tissue over the 90 days. Granulomas were observed in the liver but hepatic parenchyma remained viable and no progression or regression of the granulomatous changes in the liver was seen over the 90 days. In the brain, light microscopy did not reveal cells with CeO2 accumulation in neurites or the microvessels in the hippocampus or the cerebellum. Time-dependent oxidative stress changes were seen in the liver and spleen. Indeed, increased PC levels in liver were found at most time points (1, 7, and up to 30 days). In contrast, PC levels were decreased in the spleen at each time point examined. It cannot be excluded that these oxidative responses might have resulted from the acidic pH of nano-CeO2 suspension (i.e., 3.9). After 90 days nano-CeO2 induced anti-oxidant effects in both organs.
Hardas SS et al. further showed that even after a long-term retention time of 90 days in the liver nano-CeO2 continued to display a significant Ce(III) valence on its surface. After a single exposure, nano-CeO2 was rapidly cleared from circulating blood: Ce was weakly detected in blood from day 1 (0.20 ± 0.11% of the dose); and the Ce concentration decreased at day 7 (0.0043 ± 0.0002%) and day 30 (0.0046 ± 0.0047%), but increased at day 90 (3.81 ± 2.67%). Hardas SS et al. suggested that this increase in blood may be due to the partial dissolution of nano-CeO2 observed in the liver at that time (unpublished findings). Ce was mainly found in the liver with 21 to 40% of the nano-CeO2 dose accumulated in the reticulo-endothelial organ. The percentage of nano-CeO2 accumulated in the liver decreased over time being of 40 ± 7% at 1 h post-infusion vs. 21 ± 8% at 90 days post-infusion. Nano-CeO2 was rarely found in the brain (< 0.018 ± 0.016% of the nano-CeO2 dose).
In conclusion, the tested nano-CeO2 remained in the mammal model, mainly in reticulo-endothelial organs (i.e., liver and spleen), up to 90 days after its i.v. administration. Moreover, the particles exerted pro-oxidant effects in liver and spleen, as well as in the brain with a maximum response at 30 days post-infusion. After 90 days an antioxidant response was induced in the reticulo-endothelial organs. Hardas SS et al. mentioned that some dissolution of nano-CeO2 occurred in the liver 90 days after its i.v. administration (data not shown), that presumably released Ce ion; some of these ions could have resulted in formation of ultra-small nano-CeO2 in the liver that inherently had, due to their small size, a greater percentage of Ce(III) on their surface, the valence state that enables the antioxidant effects of nano-CeO2 (Graham UM et al., 2014), thus inducing the recovery observed at 90 days post-infusion. The results obtained in both studies suggested that the oxidative effects observed could be an adaptive response of rat organism (i.e., hormesis) as mentioned by Hardas SS et al.
Thus, Graham UM et al.(2014) investigated the in vivo transformation of nano-CeO2 in the rat liver by evaluating nano-CeO2 chemical and structural stability and solubility once sequestered inside the liver. There was no mention to any guideline or GLP. However, the study was well described and met the generally accepted scientific principles. Therefore, the study was flagged as weight of evidence.
The in-house synthesised citrate-coated nano-CeO2 (5% in water suspension) used in this study was extensively characterised (see in Table 4 above).
This nano-CeO2 suspension was i.v. infused into male SD rats (5 to 14/group) at 0 (vehicle control: water), or 85 mg/kg nano-CeO2. Animals were terminated 1 h, 20 h, 30 days and 90 days after infusion. CeO2 distribution in liver, lung, kidney, spleen, heart, thymus, and brain was by high resolution transmission electron microscopy (HRTEM) and ICP-MS. In addition, the authors characterised the nano-CeO2 content in liver using transmission electron microscopy (TEM), scanning transmission electron microscopy (STEM), EELS and HRTEM.
According to Graham UM et al., spleen and liver had sequestered approximately the same amount of nano-CeO2. The general cytoarchitecture of liver tissue was not altered by the sequestered nano-CeO2, but Kupffer cells contained many nano-CeO2 which formed small agglomerates and underwent fusion with lysosomes to form phagolysosome. Moreover, Kupffer cells with mononucleated lymphocytes had formed large granulomata in the tissue parenchyma, while surrounding hepatocytes rarely showed cytoplasmic inclusion of CeO2 agglomerates.
Nano-CeO2 formed agglomerates in the liver up to day 30, with no indication of breakdown or chemical transformation. However, after 90 days inside the liver, the i.v.-infused cube-shaped nano-CeO2 had become highly fragmented and rounded along their edges, which indicated that in vivo processing of the particles had occurred. Moreover, accumulations of copious ultrafine crystallites (1 to 3 nm) that formed in the liver within close proximity to the rounded nano-CeO2 were observed and labelled as CeO2 clouds. This represented a second generation of CeO2. EELS of the cloud particles demonstrated that the oxidation degree, when compared with the original nano-CeO2, was more reduced (38 to 70% Ce(III)). This measurable change in the valence reduction of the second-generation CeO2 could be linked to an increased free-radical scavenging potential.
Much lesser amounts of nano-CeO2 seemed to have been bioprocessed inside the spleen.
In conclusion, i.v.-infused nano-CeO2, after prolonged residence time in the liver (90 days), underwent in vivo processing. Nano-CeO2 undergoes partial dissolution inside the liver that causes a shift towards smaller particle size and an increased reactive surface area with enhanced Ce(III) activity that leads to greater free-radical scavenging potential of the ultrafines. The authors concluded that the breakdown and redistribution could be a step towards improving CeO2 biocompatibility in vivo.
Yokel RA et al. (2013) determined the biodistribution, translocation, and persistence of nano-CeO2 with various primary particle sizes in the brain, blood, liver, and spleen following a single i.v. injection to rats. The study was well documented and met generally accepted scientific principles but the test was not conducted under GLP conditions. Therefore, the study was flagged as weight of evidence.
Four size-different types of nano-CeO2 were used in this study. The different citrate-coated nanomaterials were physico-chemically characterised (see in Table 5 below). The characterisation demonstrated that the amount of stabiliser indeed influenced the agglomeration/aggregation state: the more the citrate amount was high, the less the agglomeration/aggregation tendency of nano-CeO2 was important as shown with the particle size distribution.
Table 5: Summary of the physico-chemical characteristics available on the nano-CeO2 tested by Dan M et al. (2012a) and Yokel RA et al. (2013, 2014)
Parameters |
Results |
|
|
|
Ce5 |
Ce15 |
Ce30 |
Supplier |
None (in-house synthesis) |
None (in-house synthesis) |
None (in-house synthesis) |
Synthesis method |
Hydrothermal method from cerium chloride heptahydrate |
Hydrothermal method from cerium nitrate |
Hydrothermal method from cerium nitrate |
Primary particle size |
4.6 ± 0.1 nm |
12 ± 0.2 nm |
31.2 ± 0.5 nm |
Particle size distribution (in water) |
7 nm |
25 nm |
41 nm |
Specific surface area |
121 m²/g |
71 m²/g |
15 m²/g |
Surface charge (zeta potential) in water at pH ~7.3 |
-53 ± 7 mV |
-57 ± 5 mV |
-56 ± 8 mV |
Isoelectric point |
No data |
No data |
No data |
Shape |
Polyhedral |
Polyhedral |
Cubic |
Crystallinity |
Crystalline (face-centred cubic) |
Crystalline (face-centred cubic) |
Crystalline (face-centred cubic) |
Purity / impurities |
No data |
No data |
No data |
Cerium content in nano-CeO2 suspension |
85% of the intended 5% CeO2 conc. |
70% of the intended 5% CeO2 conc. |
85% of the intended 5% CeO2 conc. |
Solubility |
No data |
No data |
No data |
Oxidation degree |
Enrichment of Ce(III) at the particle rim (surface layer) |
Enrichment of Ce(III) at the particle rim (surface layer) |
Enrichment of Ce(III) at the particle rim (surface layer) |
Surface properties |
ca. 40% surface coated with citrate |
ca. 27% surface coated with citrate |
ca. 18% surface coated with citrate |
pH of nano-CeO2 suspension |
From 7.7 to 8 |
From 7.7 to 8 |
From 7.7 to 8 |
Other information |
11.6 ± 0.3% free Ce content |
<< 1% free Ce content |
<< 1% free Ce content |
Parameters |
Ce55 |
CeM |
CeR |
Supplier |
None (in-house synthesis) |
None (in-house synthesis) |
None (in-house synthesis) |
Synthesis method |
Hydrothermal method from cerium nitrate |
Hydrothermal method from cerium nitrate hexahydrate |
Hydrothermal method from cerium nitrate heptahydrate |
Primary particle size |
55 ± 0.2 nm |
Cubic = 10 nm |
|
Rod = 2 µm |
Average diameter of 9.9 ± 2.0 nm and length of 264 ± 112 nm |
|
|
Particle size distribution (in water) |
No data |
No data |
Bimodal: 100 nm for 5% and 400 nm for 95% in 10% sucrose |
Specific surface area |
No data |
No data |
54 m²/g (estimated from diameter, length and density) |
Surface charge (zeta potential) in water at pH ~7.3 |
-32 mV |
No data |
-25 mV |
Isoelectric point |
No data |
No data |
No data |
Shape |
Polyhedral |
Cubic and rod |
Rod |
Crystallinity |
Crystalline (face-centred cubic) |
Crystalline (face-centred cubic) |
Crystalline (face-centred cubic) |
Purity / impurities |
No data |
No data |
No data |
Cerium content in nano-CeO2 suspension |
103% of the intended 5% CeO2 conc. |
No data |
No data |
Solubility |
No data |
No data |
No data |
Oxidation degree |
Enrichment of Ce(III) at the particle rim (surface layer) |
Enrichment of Ce(III) at the particle rim (surface layer) |
Enrichment of Ce(III) at the particle rim (surface layer) |
Surface properties |
ca. 15% surface coated with citrate |
No data |
ca. 100% surface coated with citrate |
pH of nano-CeO2 suspension |
From 7.7 to 8 |
From 7.7 to 8 |
No data |
Male SD rats (3 to 12/group) were i.v. infused with the different nano-CeO2 (as a 5% suspension in water) at the nominal doses of 0 (vehicle control: water), 50 (Ce55) or 100 mg/kg (Ce5, Ce15, Ce30). Animals were terminated 1, 20, or 720 h later. Blood, brain, spleen, liver, right kidney, heart, and lung samples were collected at these different time points. Ce concentration was also determined by ICP-MS in blood, brain, liver, and spleen.
The authors reported that the 15-, 30- and 55-nm nano-CeO2 were more rapidly cleared from blood as compared to the 5-nm nano-CeO2. The longer persistence of Ce5 in blood may relate to its greater extent of citrate coating and/or to its small size avoiding ready recognition by reticulo-endothelial organs. Of the different compartments/tissues studied, ≥ 98% nano-CeO2 were retained 20 h after its i.v. infusion in the reticulo-endothelial organs liver and spleen, from which there was no significant clearance over 720 h. The liver contained significantly more of the total Ce5 dose than of Ce30 at 20 h. The spleen contained significantly more of the Ce15 than the Ce5 at 30 d, suggesting preferential clearance of smaller particles by the liver, being a component of mononuclear phagocyte system (MPS). Further, Yokel RA et al. found that less than 0.5% of the Ce30 dose was excreted in the urine and faeces within 2 weeks.
Infusion of a large dose of nano-CeO2 produced modest, if not, BBB disruption, as evidenced by non-significantly increased brain fluorescein and horseradish peroxidase, not sufficient to allow appreciable brain CeO2 entry. EM revealed only occasional nano-CeO2 beyond the BBB.
These results suggested that regardless of the size or shape, nano-CeO2 mainly accumulated in reticulo-endothelial organs such as liver and spleen and primary particle size seemed to have a limited impact on the distribution. Furthermore, BBB could protect the brain from nano-CeO2 circulating in the blood since a large i.v. dose of these nanomaterials did not greatly disrupt BBB integrity whatever the primary particle size of nano-CeO2 used. Thus, brain delivery of nano-CeO2 is a challenge.
In a further publication, Yokel RA et al. (2014) aimed at determining if the biodistribution, persistence, and toxicity of nano-CeO2 were affected by dosing schedule, dose, or particle shape. The study was well documented and met generally accepted scientific principles but the test was not conducted under GLP conditions. Therefore, the study was flagged as weight of evidence.
Citrate-coated nano-CeO2 with various physico-chemical properties were selected and characterised (see in Table 5 above).
All nano-CeO2 dispersions were infused in water at a rate of 2 mL/kg rat body weight over 1 h, with the exception of the 50 mg/kg dose of nanorods, which was infused over 2.5 h.
Ce5 at 11 mg/kg, Ce30 at 6 mg/kg, and Ce rods (CeR) at 20 and 50 mg/kg were i.v. infused into male SD rats once (3 to 9/group). The Ce5 dose of 11 mg/kg was also infused daily for 5 consecutive days to male rats (5/group). Control rats were infused with the vehicle (i.e., water). Rats exposed once or repeatedly to Ce5 were sacrificed 30 days after i.v. infusion. Ce30-exposed rats were sacrificed 1 h, 30 and 90 days after i.v. infusion, while rats treated once with CeR were terminated 1 h and 30 days after i.v. infusion. Ce content was determined in multiple organs and blood using ICP-MS. The method detection limits (MDL) were 0.089 mg Ce/kg for tissues and 0.018 mg Ce/L in blood samples. Spike recovery for ICP-MS analyses was 94 ± 3%. Average relative percent difference between replicate analyses was 3.2 ± 2.0%. Ce concentrations below the MDL were assigned 50% of the MDL and included in the statistical analysis as such.
Compared to vehicle-infused controls, elevated Ce was seen in numerous tissues from CeO2-treated rats, with no evidence of entry into brain parenchyma, and that regardless of size, dose, dosing schedule, and shape of the infused nano-CeO2. According to the authors, Ce in the brain of CeO2-treated rats could be accounted for by the Ce in circulating blood. Liver, spleen, and bone marrow (MPS components), contained the largest percentage of the dose. When normalized to the dose, and compared to results of prior work with these nano-CeO2, the distribution and retention of repeated and lower doses of Ce5, Ce30 and CeR were not greatly different from much higher doses of Ce5 and Ce30. Higher doses resulted in a greater percentage of uptake by the spleen and bone marrow and a greater percentage of CeR dose in the bone marrow 30 days after its administration than the other nano-CeO2.
The authors noted that 85% of the samples, including those collected 90 days after the single nano-CeO2 administration, were above the MDL, while only 20% of the samples from non-CeO2 treated rats were above the MDL of this highly sensitive analytical method (e.g., liver, spleen, brain, bone marrow, bone, and lung).
Overall these results suggested that the biodistribution and retention of Ce after i.v. administration of different sizes, doses, dosing schedules and nano-CeO2 shapes were more similar than different.
Then, Dan M et al. (2012a) conducted an in vivo study to characterise distribution in, and clearance of nano-CeO2 from blood depending on particle size, and that following a single i.v. injection to rats. The study was well documented and met generally accepted scientific principles but the test was not conducted under GLP conditions. Moreover, there were some discrepancies regarding the number of test animals described in the "Materials & Methods" section and the figure legends. Thus, the study was flagged as weight of evidence.
The four different types of nano-CeO2 used in this study (Ce5, Ce15, Ce30, and Ce55) were the same as those detailed above in the publications of Yokel RA et al. (see in Table 5 above).
Male SD rats (3 to 21/group) were i.v. infused with the different nano-CeO2 (5% in water suspension) at nominal doses of 0 (control: water), 50 mg/kg (Ce55) or 100 mg/kg (Ce5, Ce15, Ce30). In addition, Ce ion was administered to rats at a dose level of 50 mg/kg through i.v. infusion. Blood samples were collected at 0.167, 0.5, 0.75, 1, 2, 4, 20, 168 h (1 week), or 720 h (1 month) after exposure. Blood, serum and clot distribution of Ce was assessed by ICP-MS. Animals were terminated 168 or 720 h later. Moreover, a pharmacokinetic analysis of Ce concentrations in blood was performed, notably to compare the data for nano-CeO2 to those of Ce ion.
Regarding blood distribution and clearance of nano-CeO2, Ce5 was cleared much more slowly than larger nano-CeO2. Nanomaterials larger than 5 nm were rapidly cleared from circulating blood. All nano-CeO2 seemed to display a prolonged retention throughout the rat. Traditional pharmacokinetic modelling showed best fit for 5-nm nano-CeO2 and Ce ion. After initially declining, whole blood Ce15 and Ce30 increased over 4 h after infusion; these results were not well-described by traditional pharmacokinetic modelling. The distribution of nano-CeO2 between serum and the blood clot was size-dependent. Ce ion, and Ce5 and Ce55 did not preferentially distribute into serum or clot, CeM was predominantly in the clot, and Ce15 and Ce30 migrated into the clot over 4 h, suggesting a change in surface properties of Ce15 and Ce30. The latter nanomaterial showed the greatest distribution in the clot fraction. The authors suggested that reticulo-endothelial organs may not readily recognize Ce5. Increased Ce distribution into the clot over time may be due to opsonisation.
In conclusion, traditional pharmacokinetic analysis was not very informative and nano-CeO2 pharmacokinetics were quite different from that of Ce ion. In this study conducted in 2012, some differences in distribution could be observed according to primary particle size: clearance seemed reduced for the smallest particle (5 nm). However, the highest percentage of citrate coating of this particle compared to the other ones could have influenced the behaviour of this particulate substance.
Moreover, Hardas SS et al. (2010, 2012) aimed at characterising the biodistribution of a 5-nm nano-CeO2 suspension from blood into brain up to 30 days after systemic administration. The study was well documented and met generally accepted scientific principles but the test was not conducted under GLP conditions. Therefore, the study was flagged as weight of evidence.
A water or saline suspension (4.35%) of citrate-coated nano-CeO2 (8.7 x 10E16 particles/mL) was used in this study. The in-house synthesised nano-CeO2 was extensively characterised (see in Table 4 above).
In a first study (2010), the nano-CeO2 suspension was i.v. infused at nominal doses of 0 (control), 100, 175, or 250 mg/kg into male SD rats (7 to 12/group), which were terminated after 1 or 20 h. In the second study (2012), the nano-CeO2 suspension was i.v. infused at measured doses of 0 or 85 mg/kg into male SD rats (7 to 9/group) which were terminated 30 days later. CeO2 concentration, localization, and chemical speciation in the brain, blood, liver and spleen were assessed by ICP-MS, LM and EM, and EELS. Na fluorescein and horseradish peroxidase (HRP) were given i.v. as BBB integrity markers.
In the first study, mortality was seen after administration of 175–250 mg CeO2/kg. However, given i.v. at 100 mg/kg (nominal dose), no systemic effect and no significant differences in organ weight were found between CeO2-infused and control rats, except for the spleen. CeO2 accumulation was seen in liver and spleen tissues, but not in the brain. Indeed, 20 h after infusion of 100 mg CeO2/kg, brain HRP was globally unchanged, showing a probable lack of BBB penetration. EM and EELS revealed a high Ce(III) valence in nano-CeO2 agglomerates in the brain vascular compartment. Nano-CeO2 was not seen in microvascular endothelial or brain cells. These results suggested that in the brain, most of the nano-CeO2 was located on the luminal side of the BBB endothelial cells.
In the second study, the BBB was visibly intact and no nano-CeO2 was seen in the brain cells at day 30 post-infusion. The particles located on the luminal side of the BBB endothelial cells. Moreover, as observed in the previous study, nano-CeO2 was found accumulated in peripheral organs like the liver. EELS analysis showed that even after a long-term exposure time of 30 days nano-CeO2 continued to display a significant Ce(III) valence on the surface.
In conclusion, nano-CeO2 remained in the mammal model, mainly in reticulo-endothelial organs, up to 30 days after its i.v. administration but did not cross the BBB.
In a further publication, Dan M et al. (2012b) investigated the potential association of a 5-nm nano-CeO2 suspension with brain capillary cells and/or the possible crossing of the BBB when administering the particles by in situ perfusion into rat brain. The study was well documented and met generally accepted scientific principles but the test was not conducted under GLP conditions. Thus, the publication was flagged as supporting study.
Dan M et al. selected the same in-house synthesised nano-CeO2 as those used by Hardas SS et al. in their studies from 2010 and 2012 (see in Table 4 above).
Nano-CeO2 uptake in the brain of male SD rats (3 to 10/group) was determined using the in situ brain perfusion technique at 15 and 20 mL/minute flow rates; 30, 100, and 500 µg/mL CeO2 perfused for 120 seconds at 20 mL/minute; and 30 µg/mL perfused for 20, 60, and 120 seconds at 20 mL/minute (n = 3 to 6 animals per group). Eight brain regions and a choroid plexus (ipsilateral to the perfusion) were collected. The capillary depletion method and LM and EM were used to determine CeO2 capillary cell and brain parenchymal association and localization.
The vascular space was not significantly affected by brain perfusion flow rate or nano-CeO2, demonstrating that again nano-CeO2 did not influence BBB integrity. Measurements performed using ICP-MS showed a trend towards increasing Ce concentration in the choroid plexus in the 100 and 500 µg/mL CeO2 perfusion groups. Whereas Ce uptake was not significantly modulated in the other eight brain regions. CeO2 uptake into the eight brain regions was similar after 120-second perfusion of 30, 100, and 500 µg CeO2/mL. However, a trend towards increasing CeO2 uptake space was found in the eight brain regions and choroid plexus after 60 versus 20 seconds, and this parameter was similar after 60 and 120 seconds. The capillary depletion method showed that less than 0.4% of the perfused nano-CeO2 dose was associated with cerebral capillaries and brain parenchyma. Moreover, 99.4 ± 1.1% of the perfused nano-CeO2 was found in the brain associated with the capillary fraction. EM showed that nano-CeO2 located on the endothelial cell luminal surface.
In conclusion, when administered by in situ perfusion into brain, nano-CeO2 predominantly localized on the surface of the brain capillary cells and very little nano-CeO2 passed through the endothelial cells into brain extracellular fluid or brain cells.
Molina RM et al. (2014)/Konduru et al. (2015) investigated the bioavailability, tissue distribution, clearance and excretion of radioactive 141Ce after i.v. injection of neutron-activated 141nano-CeO2 in rats. There was no mention to any guideline or GLP. However, the studies were well documented and met the generally accepted scientific principles. Thus, the studies were awarded a reliability score of 2 (Klimisch, 1997) and were flagged as supporting study.
As described previously, the commercial nano-CeO2 (NM-212) powder or the in-house synthesized nano-CeO2 were neutron activated; the process generated the radioisotope 141Ce. The physico-characterisation was performed on non-radioactive nanoparticles, thus supposing that radioactive nano-CeO2 had the same physico-chemical properties as its non-radiolabelled counterpart (see in Table 4 above).
In both studies, male Wistar rats (5/group) were exposed once to 0 (control) or 1 mg/kg of nano-CeO2 by i.v. route. All treated rats were sacrificed 2 h and 2 days post-administration. Then, the following tissue samples were collected: urine, faeces, blood, plasma, serum, lung, bone marrow, femoral bone, skin, brain, skeletal muscle, testicles, kidneys, spleen, heart, liver, stomach, small intestine, large intestine, and caecum. Moreover, 24-h samples of faeces and urine were collected at 24 h post-dosing. The Ce content in the samples was determined by measuring the radioactivity (141Ce) using a gamma counter.
At 2 h, nanoparticulate 141Ce was retained in the liver (79 to 87 % of the dose), spleen (ca. 4-5%) and bone (< 2%), all organs that typically remove circulating particles. No redistribution was observed in the 141nano-CeO2 group 2 days after. The faecal and urine excretion of 141Ce in the nanoparticle group during the first 24 h was very low (0.08 - up to 5.8% and < 0.02%, respectively).
In conclusion, in both studies, nano-CeO2 was immediately taken up in organs rich in mononuclear phagocytes with direct access to the circulating blood, such as those in the liver, spleen and bone.
In addition, Rojas S et al. (2012) explored the biodistribution of a surface-modified nano-CeO2 in the organs of living rodents up to 2 h after i.v. injection. The study displayed numerous restrictions: only 5 animals were used in this study; there was no data on aggregate/agglomerate state of the particles; the CeO2 dose administered to rats and the nanoparticle excretion were not clearly documented; no statistical analysis was performed. Thus, the study was awarded a reliability score of 3 (Klimisch, 1997) and was flagged as supporting study.
In-house synthesised nano-CeO2 was used in this study. The nanoparticles were covalently radiolabelled with [18F]fluorobenzoyl after NH2 functionalisation. Only the non-radiolabelled/non-functionalised particles were characterised (see in Table 4 above); the authors thus assumed that the radiolabelled counterpart displayed similar physico-chemical properties.
Male SD rats (5/group) received an i.v. bolus injection of 18F-NH2-nano-CeO2. Doses administered were 4.8 ± 3.1 MBq resuspended in 0.5 mL of saline. The biodistribution was studied in vivo by positron emission tomography (PET) immediately after the injection and up to 120 min post-i.v. Moreover, the biodistribution was also examined ex vivo by measuring the radioactivity in each collected organ from animals sacrificed at the end of the PET acquisition (e.g., bladder, lung, liver, spleen, kidney, intestine, blood, brain).
Further, the level of 18F-NH2-nano-CeO2 urinary excretion was determined by measuring the radioactivity in a gamma-counter. Rojas S et al. also assessed the nano-CeO2 metabolization in plasma at 0, 30, 60, 90, and 120 minutes, by measuring the radioactivity in aliquots of centrifuged supernatant and non-centrifuged samples in a gamma-counter.
As soon as 18F-NH2-nano-CeO2 entered the blood, the particles became trapped in organs such as the lungs, spleen, and liver. Time-activity curves showed that the concentration observed in these organs for the initial frames of the study remained almost constant until the end of the imaging study. Moreover, the low radioactivity content observed in the intestine indicated that despite the accumulation observed in the liver, 18F-NH2-nano-CeO2 was not excreted through the biliary tract. The progressive increase of radioactivity in the urinary system could indicate that the fraction of 18F-NH2-nano-CeO2 that was not phagocytised and circulated in the bloodstream was excreted through kidney into urine.
In addition, according to the authors, 18F-NH2-nano-CeO2 was progressively metabolized in plasma, and the radioactivity found in urine corresponded to soluble metabolites of 18F-NH2-nano-CeO2 and not to intact nanoparticles.
Results of the ex vivo study confirmed the results obtained in vivo in all the organs evaluated and indicated that after 2 h, almost all 18F-NH2-nano-CeO2 was cleared from blood. The brain presented the lowest radioactivity content among the evaluated organs but this concentration was similar to that of blood. However, given the low radioactivity found in the brain, it cannot be ruled out that this cerebral content might result from a contamination of brain samples during animal sacrifice and tissue collection alternatively, that the particles could remain trapped in the vascular endothelium of the brain. Moreover, since nano-CeO2 are known to be insoluble, rarely found in urine and rather eliminated through faeces, it cannot be excluded that the radioactivity detected by Rojas S et al. in urine might have resulted from 18F (± NH2) groups released from the nanoparticles due to unknown reactions/enzymes breaking the covalent bonds (e.g., OH bonds) between 18F and/or NH2 groups and nano-CeO2.
Given the weak number of animals used in this study, the lack of characterisation of radiolabelled NH2-nano-CeO2, insufficient data regarding the excretion, and unclear documentation on the CeO2 dose administered, these results should be taken with caution.
Through two publications (2009, 2011), Hirst SM et al. investigated the distribution of nano-CeO2 in i.v. exposed mice. The studies were considered to meet generally accepted scientific principles. However, there were some restrictions regarding the level of details on test animals (e.g., source, age, weight) and the physico-chemical characterisation of nano-CeO2; but, at the publication date, the scientific community required less physico-chemical endpoints in such articles. Nevertheless, although described in "Materials and Methods" section, toxicokinetic data on control animals were not provided in the publication. Thus, it was difficult to assess the biological relevance of CeO2 distribution in investigated tissues. Moreover, there was no data available regarding the experimental validation of the detection method, ICP-MS (e.g., limit of detection). Thus, the study was awarded a reliability score of 3 (Klimisch, 1997) and was flagged as supporting study.
The physico-chemical characteristics of the in-house synthesised nano-CeO2 used in this study are displayed in Table 4 above. To perform a real-time biodistribution, nano-CeO2 was functionalised by conjugating carboxyfluorescein.
In the first study (2009), C57BL mice (4/group) mice were i.v. infused with either sterile PBS or a nano-CeO2 suspension (in PBS). On day 1, group 1 received a low dose (0.1 mg/kg) of nano-CeO2, group 2 received a high dose (0.5 mg/kg), and the remaining control mice received PBS. Seven days post injection, 4 control mice, 4 low-dose mice, and 4 high-dose mice were necropsied and examined. The remaining mice received the same injection as day 1 on day 15. Remaining mice were then necropsied and organs harvested on day 30.
In the second study (2011), female CD-1 mice (at least 3/group) were i.v. dosed once a week for 2 or 5 weeks with 0 (control: PBS) or 0.5 mg/kg nano-CeO2. Animals were sacrificed one week after the last administration.
An histological examination of major organs (brain, lungs, liver, spleen, kidneys, and pancreas) was performed using LM and EM. Nano-CeO2 distribution was evaluated in organs using LM, EM, IHC and ICP-MS. Moreover, immune responses were investigated by counting white blood cells.
In the first study, i.v. administered nano-CeO2 remained deposited in tissues (e.g., liver, spleen, and kidneys) at least 30 days after injection. Moreover, C57BL mice receiving twice the highest nano-CeO2 dose (0.5 mg/kg) did not experience any tissue damage up to at least 30 days after administration.
In the second study, the remaining nano-CeO2 was deposited in the spleen (ca. 34 ± 11 µg CeO2/g organ) followed by the liver (ca. 25 ± 5 µg/g), and traces were found in the lungs or in the kidneys (< 1 µg/g). However, there was no distribution of nano-CeO2 in the heart and brain of any i.v. infused mice (< 0.1 µg/g). Nano-CeO2 did not cross the BBB. Moreover, the authors claimed that mice excreted nano-CeO2 through faeces, but not via urine.
Nano-CeO2 showed no overt toxicity and no relevant induction of immune responses.
In conclusion, in this model using mice, the tested nano-CeO2 displayed the classical behaviour of poorly soluble particles after i.v. injections: low toxicity, faecal excretion but biopersistence in reticulo-endothelial organs such as liver and spleen which are involved in the clearance of foreign materials.
Discussion: intravenous route
All published studies demonstrated, overall, that nano-CeO2 administered by i.v. route was rapidly translocated from blood into reticulo-endothelial organs such as the spleen and liver in which it can be retained for a long time, and that regardless of size, shape or surface treatment of the tested nano-CeO2 and whatever the species (rats or mice) used. Thus, even though nano-CeO2 was generally administered in massive doses directly in animal blood, the tested nanomaterials showed a weak retention in blood. However, the blood retention time seemed influenced by some physico-chemical characteristics such as size and/or citrate-coating amount which might all influence nano-CeO2 interactions with blood proteins. Indeed, citric acid coating of nano-CeO2 was reported to increase blood circulation time (Walkey C et al., 2015). Moreover, citric acid-coated nano-CeO2 was found in blood circulation up to 30 days post-administration, and longer blood circulation times were observed for smaller nanoparticles (5 nm, with the highest amount of citrate coating) as compared to larger nano-CeO2 (Yokel RA et al., 2014). In addition, the major distribution of nano-CeO2 in spleen and liver tissues was also described by Walkey C et al. (2015): in vivo real-time imaging revealed that i.v. injection of carboxy-fluorescence conjugated nano-CeO2 (F-nano-CeO2) deposited in liver within 3 h of injection. Moreover, F-nano-CeO2 was mainly found deposited in the liver, spleen and lungs and persisted 2 weeks after F-nano-CeO2 injection (Walkey C et al., 2015). The studies of Dan M et al. (2012a) and Yokel RA et al. (2013) demonstrated that smaller nano-CeO2 (< 5 nm) could be preferentially accumulated in the liver rather than in the spleen.
It has to be noted that, even though the nanoparticles were systemically available, nano-CeO2 did not accumulate in the brain and did not alter the BBB integrity of i.v. infused rats in any of the studies detailed above. Moreover, even when the nanoparticle administration directly targeted the brain, nano-CeO2 was only found localized on the endothelial cell luminal surface.
In all studies mentioned above i.v. injected nano-CeO2 was found to be very poorly eliminated from reticulo-endothelial organs even 90 days after i.v. injection and that this weak excretion occurred mainly through faeces.
There were two studies focusing on the metabolization of nano-CeO2 administered by i.v. route. Rojas S et al. (2012) demonstrated that functionalised/radiolabelled nano-CeO2 i.v. administered to rats was progressively metabolized in plasma, and that the increased radioactivity found in urine corresponded to soluble metabolites of nano-CeO2 and not to intact nanoparticles. However, the radioactivity detected in urine was considered to most likely result from 18F (± NH2) groups released from the nanoparticles due to unknown reactions/enzymes breaking the covalent bonds (e.g., OH bonds) between 18F and/or NH2 groups and nano-CeO2. Nevertheless, it seemed that nano-CeO2 underwent partial dissolution inside the liver (but less in the spleen) with time; this dissolution caused a shift towards smaller particle size with a shift from a Ce(IV) to Ce(III) enrichment that leads to greater free-radical scavenging potential. Indeed, in their numerous studies, Yokel RA et al. demonstrated that 5-nm nano-CeO2 underwent a Ce(III) enrichment. Then, a 30-nm nano-CeO2 showed similar Ce(III) enrichment on its surface while its core had a greater percentage of Ce(IV) (Tseng MT et al., 2014; Yokel RA et al., 2012). Moreover, Graham UM et al. (2014) described a biotransformation of 30-nm CeO2 occurring after prolonged residence time in the liver. In this study, the authors observed that nano-CeO2 underwent in vivo processing after 90 days. This dynamic transformation included a particle-size effect towards ultrafines (1 to 3 nm; second-generation CeO2), combined with a measurable change in the valence reduction (38 to 70% Ce(III)) and redox activity. The authors hypothesized that the liver environment promoted the processing of nano-CeO2 through its localized liver acid-base physiology; cellular pH is tightly regulated and nano-CeO2 would primarily encounter a low pH in lysosomes. In vivo processing of nano CeO2 over time was also observed in mice liver and lung by Modrzynska J et al. (2018) using single particle ICP-MS. In this study, female C57BL/6 mice received either via intravenous injection or via intratracheal instillation a single dose of 162 µg nano CeO2 (primary particle size: 13.0 +/- 12.1 nm, mean size distribution diameter: 35 nm) and the retention of NP was measured at 1, 28 and 180 days after exposure. In both experiments (i.v. and i.t. exposures), single particle ICP-MS showed that the size of the CeO2 NP decreased over time in both organs.
In addition, it could be observed from the studies described above that i.v. administered nano-CeO2 showed few or no adverse effects and rare mortality in rodents exposed to relatively large doses (mainly ~100 mg/kg; up to 750 mg nano-CeO2/kg) up to 90 day after i.v. infusion. Moreover, the primary particle size, the shape as well as specific surface area of nano-CeO2 had a limited impact on the systemic toxicity. Some histopathological lesions were described in liver of exposed rats: some hepatocyte degeneration; non-necrotizing granulomata formation which persisted without great resolution or progression up to 90 days after dosing and without progression to frank hepatic necrosis. However, no evidence of liver pathology was observed.
Overall, these data demonstrate that i.v. injected nano-CeO2 is mainly accumulated in liver and spleen of rodents, two organs known for their role in elimination of foreign agents, and that regardless of the physico-chemical characteristics or the doses administered. In contrast, nano-CeO2 is rarely observed in the brain and no damage of the BBB is evidenced, even when the nanoparticle administration directly targeted the brain. Nano-CeO2 could undergo partial dissolution inside the liver and spleen ; this might cause a shift towards smaller particle size and an increase reactive surface area with enhanced Ce(III) activity that leads to greater free-radical scavenging potential.
Intraperitoneal injection
There are 2 articles available on nano-CeO2 toxicokinetic behaviour in rodents exposed by i.p. route (Hirst SM et al., 2011; Rocca A et al., 2015).
First, Rocca A et al. (2015) investigated the antioxidant effects of nano-CeO2 as a potential pharmaceutical approach for the treatment of obesity. In this context, the authors evaluated the biodistribution of nano-CeO2 following repeated i.p. administration to rats. There was no mention to any guideline or GLP. Although the study was considered to meet generally accepted scientific principles, there were some restrictions: no data on the exact number of animals observed for histopathology and for CeO2 biodistribution assessment, no data on whether analysis was performed on wet or dry tissues. Thus, the study was awarded a reliability score of 2 (Klimisch, 1997) and was flagged as supporting study.
A commercial nano-CeO2 (5 to 80 nm) from Sigma was used in this study. The nanomaterial was characterised in a previous study (Ciofani G et al., 2013). The physico-chemical data are displayed in Table 6 below.
Male Wistar rats (6/group) were i.p. injected with nano-CeO2 at a single dose of 0 (control) or 0.5 mg/kg body weight twice a week for 6 weeks. At the end of exposure period, visceral fat, brain, liver, kidney, spleen were collected. Nano-CeO2 content was determined by using an ICP-AES. Histological analysis of visceral fat, liver, kidney, and spleen was performed on tissues stained with hematoxylin and eosin (H&E) and using LM. In addition, all animals were weighted once a week during the 6-week exposure period.
Nano-CeO2, i.p. injected in Wistar rats, did not have appreciable toxic effects, but instead efficiently contributed in reducing the weight gain. Concerning organ accumulation, Rocca A et al. estimated an accumulation of about 3% of the whole injected amount of nano-CeO2 in the examined organs and nano-CeO2 was mainly accumulated in the spleen at the end of the 6-week treatment. Despite the strong accumulation in the spleen, histological analysis with H&E did not reveal any impairment of the tissues. Furthermore, analysis on other organs (liver, kidney, and adipose tissue) showed a typical morphology of tissues without difference between treated and control rats. Given the i.p. administration, the authors expected that a consistent amount of nano-CeO2 remained in this compartment. Concerning the excretion of nano-CeO2, Rocca A et al. did not perform analysis on faeces and/or urine.
Table 6: Summary of the physico-chemical characteristics available on the various nano-CeO2 tested by intraperitoneal route
Parameters |
Hirst SM et al. (2011) |
Rocca A et al. (2015) |
Supplier |
None (in-house synth.) |
Sigma |
Synthesis method |
Wet chemistry method |
No data |
Primary particle size |
3 to 5 nm |
5 to 80 nm |
Particle size distribution |
10 to 50 nm |
92% peak at a size of ca. 230 nm |
Stability |
Agglomeration |
Agglomeration / aggregation |
Specific surface area |
No data |
No data |
Surface charge (zeta potential) |
No data |
ca. -15 mV |
Isoelectric point |
No data |
No data |
Shape |
No data |
No data |
Crystallinity |
Crystalline (fluorite) |
Crystalline (cubic face-centred lattice) |
Purity / impurities |
No data |
Impurities: < 1% of atom content |
Solubility |
No data |
No data |
Oxidation degree |
Mixing of Ce(III) and Ce(IV), with a relatively higher abundance of Ce(III) |
ca. 23% Ce(III) content, with coexistence of Ce(III) and Ce(IV) oxidation degrees |
Surface properties |
No data |
No data |
pH of nano-CeO2 suspension |
≤ 3.5 |
No data |
Photocatalytic activity |
No data |
No data |
Other information |
No data |
Antioxidant capacity |
Then, Hirst SM et al. (2011) investigated the distribution of nano-CeO2 in i.p. exposed mice. There was no mention to any guideline or GLP. Although the study was considered to meet generally accepted scientific principles, there were some restrictions regarding the level of details on test animals (e.g., source, age, weight) and on the physico-chemical characterisation of nano-CeO2 or regarding missing toxicokinetic data on control animals. Thus, it was difficult to assess the biological relevance of CeO2 distribution in investigated tissues. Therefore, the study was awarded a reliability score of 3 (Klimisch, 1997) and was flagged as supporting study.
The in-house synthesised nano-CeO2 was characterised; the physico-chemical properties are summarised in Table 6 above.
Female CD-1 mice (3/group) were dosed once a week for 2 or 5 weeks with 0 (vehicle control: PBS) or 0.5 mg/kg nano-CeO2 by i.p. route. Animals were sacrificed one week after the last administration. A histological examination of major organs (brain, lungs, liver, spleen, kidneys, and pancreas) was performed using LM and EM. Nano-CeO2 distribution was evaluated in organs using LM, EM, IHC and ICP-MS. Moreover, immune responses were investigated by counting white blood cells.
In i.p. injected animals, the deposition was greatest in the spleen followed by the liver, and traces were found in the lungs or kidneys. However, there was no distribution of nano-CeO2 in the heart and brain of any exposed mice. Nano-CeO2 did not cross the BBB. The authors claimed that mice eliminated nano-CeO2 by excreting the nanoparticles through faeces but the corresponding data were not shown.
In addition, nano-CeO2 showed no overt toxicity and no induction of immune responses.
In conclusion on the intraperitoneal route, both nano-CeO2 tested by Hirst SM et al. (2011) and Rocca A et al. (2015) in mice and rats, respectively, displayed a similar behaviour: low toxicity and distribution in reticulo-endothelial organs such as spleen mainly or liver which are involved in the clearance of foreign materials.
Intravitreal injection
There was 1 study available on nano-CeO2 toxicokinetic behaviour in rodents exposed by intravitreal route (Wong LL et al., 2013).
Wong LL et al. (2013) aimed at determining the temporal and spatial distributions of nano-CeO2 after a single intravitreal injection in healthy rat eyes. There was no mention to any guideline or GLP and several restrictions such as no clear documentations on the number of animals per group and on the results. Thus, the study was awarded a reliability score of 3 (Klimisch, 1997) and was flagged as supporting study.
The tested CeO2 particles were in-house synthesised using a simple wet chemistry method. Nano-CeO2 was poorly characterised in this study and most of the characteristics presented here came from another publication (Zhou X et al., 2011). According to Wong LL et al., the water-suspended nanoparticles had a size of 3-5 nm being stable in a wide range of pH buffers and upon aging. However, in a previously study (Zhou X et al., 2011), the particles were found to form aggregates of up to 294 nm in saline solution. At the concentration of 1 mM in saline, the zeta potential value was of +10 ± 3 mV. In addition, according to Zhou X et al. (2011), nano-CeO2 had a Ce(III) oxidation degree.
SD albino rats (2 to 5/group) were intravitreally injected 2 µL of saline (control) or nano-CeO2 suspension at concentrations ranging from 1 µM (0.344 ng) to 1 mM (344 ng). Animals were sacrificed at 1 h, 21, 60, 90 and 120 days post-injection for collection of ocular samples. Nano-CeO2 biodistribution was measured at the different time points in whole eyes or in eyes that had been dissected into component parts (i.e., retina, lens and rest of eye cup) using ICP-MS. Furthermore, effects of nano-CeO2 on retinal morphology and function were measured.
Nano-CeO2 was rapidly taken up by the retina (from 1 h after injection) and preferentially retained in this tissue even after 120 days. Moreover, the nanoparticles were not actively eliminated from the eye, with an elimination half-life of 525 days in the eye and 414 days in the retina. Nano-CeO2 did not induce any acute or long-term negative effects on retinal function or cytoarchitecture.
CONCLUSIONS
Absorption
From the results mentioned above, > 90 - 99% of the administered dose was found in the faeces after oral administration and < 0.01% was found in organs suggesting that nano CeO2 was hardly absorbed through the GI. Further, a dermal absorption and systemic distribution of nano-CeO2 is not expected from intact skin according to Mauro M et al. 2019 who used an in vitro model of dermal penetration. After inhalation or intratracheal instillation, it can be evaluated that nano-CeO2 was mainly deposited and retained in lungs and lung associated lymph nodes, was weakly absorbed from lungs to the blood, and quickly but poorly distributed from the blood to extrapulmonary tissues. Indeed, < or = to around 1% of nano-CeO2 was found in organs excluding skeleton from the studies performed by Tentschert J et al. (2020), Geraets L et al. (2012) and He X et al. (2010), although intratracheal instillation induced a higher deposition of nano-CeO2 in the lungs (~80%) than in the inhalation study (< 13%) and ~ 0,3 up to an estimated 1,2 % was found in skeleton (Tentschert J et al. (2020) and Molina RM et al. (2012).
Further, based on the percentage of nano-CeO2 doses in different organs after intragastric administration compared to i.v. exposure, Boyes WK et al. (2012) found that from 0.00001% (for spleen, lung, heart, testicles, and brain) to 0.01% (for liver) of the nano-CeO2 administered intragastrically was absorbed in agreement with the abovementioned studies, then translocated to and was retained in the liver, spleen, lung, or kidney after 1 week (He X et al., 2010; Yokel RA et al., 2012). Furthermore, Boyes WK et al. (2012) calculated that around 0.1 to 1% of 7-nm CeO2 particles introduced by i.t. route were absorbed from the lung and translocated to peripheral organs after 1 month, based on a comparison of the percentage of nano-CeO2 doses in different organs after i.t. exposure to i.v. administration (He X et al., 2010; Yokel RA et al., 2012). Although the routes of administration, the nanoparticle characteristics and the doses/concentrations were different in the studies presented above, all data showed that nano-CeO2 was barely found in blood, suggesting a poor absorption or a rapid distribution.
Nano-CeO2 is an inorganic substance with a really low solubility in various media (Andreescu D et al., 2014; Brunner TJ et al., 2006; He X et al., 2010; Keller J et al., 2014; Molina RM et al., 2014/ Konduru N et al. 2015; Van Hoecke K et al., 2009; Yokel RA et al., 2014) and agglomeration/aggregation was found in almost if not all studies. Thus, this could explain, at least partially, the limited absorption and the apparent quite similar distribution observed in the abovementioned studies, whatever the routes of exposure.
Moreover, in spite of the administration of large doses, little or no sign of systemic toxicity was found in exposed rats whatever the route of exposure, supporting the low absorption observed.
These observations thus suggest that nano-CeO2 absorption could be extremely low.
Furthermore, CeO2 nano- and micro-particles seem to behave similarly in terms of absorption since in both cases rare mortality and no systemic toxicity were observed in the different rodent models, regardless of the route of administration.
Overall, these data demonstrate that whatever the route of administration (oral, dermal, inhalation, i.t., i.v., or i.p.) nano-CeO2 is poorly absorbed.
Absorption rate – oral: < 0.1%
Absorption rate – dermal: < 0.1%
Absorption rate – inhalation: < 3%
Distribution
According to the abovementioned studies, regardless of the rodent model, routes of administration, nanoparticle characteristics or doses, nano-CeO2 was mainly distributed in liver, spleen and to a lower extent in lungs in which accumulation can occur. It has to be noted that as described above and further documented by Boyes WK et al. (2012) around 1 % or less of the administered dose is generally absorbed after lung or GI exposure. Therefore, as nano-CeO2 absorption through blood and GI barriers is supposed to be weak, the amount of distributed nano-CeO2 is expected to be low. Skeleton was also found to retain nanoCeO2 in a 2 -year inhalation study and an estimated quantity of around 1 % was calculated from femur and bone marrow contents in this tissue by Tentschert J et al. (2020).
Interestingly, nano-CeO2 was not observed in rodent brain, indicating that these nanoparticles could not cross the BBB. These observations are not surprising given the well-known restrictive properties of this barrier. Moreover, even though the nose-to-brain transport (i.e., olfactory nerve) of exogenous materials is a potential route for bypassing the BBB (Oberdörster G et al., 2009), the inhalation studies of Geraets L et al. (2012) and Tentschert J et al. (2020) showed very limited accumulation of nano-CeO2 in rodent brain, indicating that this nose-to-brain route might be inefficient in the case of nano-CeO2. Further, it should be noted that the particle retention in lungs is much shorter in rodent lungs and declines much faster than it does in human; the transport rate (via macrophage-mediated clearance) is 10 times higher in rodents than in other species (Kreyling G et al., 2013). This suggests that nano-CeO2 distribution could be even lower in human subjects than that observed in murine models.
In conclusion, only a weak systemic distribution of nano-CeO2 is expected, with a small but major accumulation in skeleton and reticulo-endothelial organs such as liver and spleen. The nanoparticles would rather accumulate and persist at the site of deposition, a typical behaviour of poorly soluble and inorganic particles.
Metabolization
Regardless of the route of administration, there were too low data available to fully assess the metabolization of nano-CeO2. The few results available came from studies performed on rodents exposed by i.v. route (Graham UM et al., 2014; 2018; Rojas S et al., 2012) or after inhalation (Tentschert J et al. (2020). Microscopic finding in the hepatic metabolizing tissue and in the spleen, potentially illustrative of metabolic activity, were seen following acute exposure to nano-CeO2 by i.v. route at a dose of 85 mg/kg in male rats (Graham UM et al., 2014; 2018). The authors found that i.v.-infused nano-CeO2, after prolonged residence time in the liver and in the spleen(90 days), underwent in vivo processing. This dynamic transformation consisted in a partial dissolution inside the liver with time that caused a shift towards smaller particle size (1 to 3 nm) with a shift from a Ce(IV) to Ce(III) enrichment (38 to 70% Ce(III)) that leads to greater free-radical scavenging potential. The authors hypothesized that the liver environment promoted the processing of nano-CeO2 through its localized liver acid-base physiology; cellular pH is tightly regulated and nano-CeO2 would primarily encounter a low pH in lysosomes. In the spleen, less dissolution of nanoceria was observed but conversion to cerium (III) phosphate nanoneedles indicative of a precipitation mechanism involving Ce ion and ion transport (from liver) instead (Graham UM et al., 2018). The authors suggested that Ce-phosphate nanoneedle formation in spleen may counteract the toxicity caused by free Ce ions derived from dissolving (bioprocessing) nanoparticles. In vivo processing of nano CeO2 over time, as a decrease in particle size, was also observed in mice liver and lung by Modrzynska J et al. (2018) after both intravenous injection or intratracheal instillation of a single dose of nano CeO2. In another study, Rojas S et al. (2012) investigated nano-CeO2 metabolization in SD rats i.v. injected with functionalised (NH2 groups) and radiolabelled (18F groups) nano-CeO2. The authors claimed that the functionalised/radiolabelled nano-CeO2 was progressively metabolized in plasma, and that the increased radioactivity found in urine corresponded to soluble metabolites of nano-CeO2 and not to intact nanoparticles. Since nano-CeO2 is described as insoluble like its micrometric counterpart, a metabolization of these nanoparticles in plasma seems unlikely. Thus, the radioactivity detected in urine most likely resulted from 18F (± NH2) groups released from the nanoparticles due to unknown reactions/enzymes breaking the covalent bonds (e.g., OH bonds) between 18F and/or NH2 groups and nano-CeO2. Tentschert J et al. (2020), using imaging mass spectrometry, have observed CeO+ ions and cerium phosphate ions in the femur sections suggesting that particle dissolution of CeO2 and recombination with phosphates have occured either directy in the bone or on the on way between lung to bone.
A plasma metabolization of nano-CeO2 seems further unlikely given the weak retention time of nano-CeO2 in blood as demonstrated for instance by Yokel RA et al. (2009) with a half-life value (t½) of 7.5 min. Nevertheless, like its micrometric counterpart, nano-CeO2 as an inorganic compound is not expected to undergo significant metabolism.
Elimination
When absorbed, nano-CeO2 was poorly eliminated regardless of the rodent model, routes of administration, nanoparticle characteristics or doses. Data rather illustrated a prolonged retention of the insoluble nano-CeO2 in a loco-regional manner (i.e., where deposited) and, secondarily, in reticulo-endothelial tissues like the liver or the spleen and skeleton. Following intratracheal instillation, the clearance rate of nano-CeO2 from lungs was estimated to be low with an elimination half-life ranging from 103 to 140 days (He X et al., 2010; Molina RM et al., 2014). However, elimination of nano-CeO2 occurred, notably when nanoparticles were orally administered; the major part (> 90 to 99%) was excreted through faeces while urinary excretion is negligible (0.005%) (He X et al., 2010; Hirst SM et al., 2011; Molina RM et al., 2014/Konduru N et al., 2015). Fecal excretion that could be in the range of some hundred of µg/day and urinary excretion have also been reported after inhalation exposure by Tentschert et al. (2020) and Molina et al., (2014)/Konduru et al., (2015).
In addition, it seems that elimination behaviour did not differ between nano- and micro-CeO2; both are mainly excreted through faeces in murine models exposed notably via oral route.
Overall, nano-CeO2 shows a low absorption, loco-regional deposition and/or low distribution in reticulo-endothelial organs and skeleton, no or few metabolization but partial dissolution instead, and an excretion occurring mainly through faeces whatever the routes of exposure. Varying the physico-chemical characteristics of nano-CeO2 had no or rare impact on the toxicokinetic behaviour of the particles, suggesting that the determinant characteristics are rather the chemical composition and/or the solubility than the primary particle size or the specific surface area. It has to be noted that the nano-CeO2 tested in the abovementioned studies were frequently described as agglomerated/aggregated, regardless of the preparation used. Therefore, these agglomerated/aggregated nanomaterials might have displayed similar behaviours in terms of toxicokinetic. Nevertheless, the studies of Yokel RA et al. demonstrated that the biodistribution of nano-CeO2 coated with a stabilizer was generally similar to that observed for non-coated nano-CeO2.
Additional REFERENCES
Modrzynska J, Berthing T, Ravn-Haren G, Kling K, Mortensen A, Rasmussen RR, Larsen EH, Saber AT, Vogel U, Loeschner K. (2018). In vivo-induced size transformation of cerium oxide nanoparticles in both lung and liver does not affect long-term hepatic accumulation following pulmonary exposure.PLoS One. 13(8):e0202477.
Li D, Morishita M, Wagner JG, Fatouraie M, Wooldridge M, Eagle WE, Barres J, Carlander U, Emond C, Jolliet O. (2016). In vivo biodistribution and physiologically based pharmacokinetic modeling of inhaled fresh and aged cerium oxide nanoparticles in rats.Part Fibre Toxicol. 13(1):45.
Walkey C, Das S, Seal S, Erlichman J, Heckman K, Ghibelli L, Traversa E, McGinnis JF and Self WF. (2015). Catalytic properties and biomedical applications of cerium oxide nanoparticles. Environ.Sci.: Nano., 2, 33.
Arts JHE, Hadi M, Irfan MA, Keene AM, Kreiling R, Lyon D, Maier M, Michel K, Petry T, Sauer UG, Warheit D, Wiench K, Wohlleben W, Landsiedel R. (2015)A decision-making framework for the grouping and testing of nanomaterial (DF4nanoGrouping).Regulatory Toxicology and Pharmacology 71: S1–S27.
Boyes WK, Chen R, Chen C, Yokel RA. (2012). The neurotoxic potential of engineered nanomaterials.Neurotoxicology,33(4):902-10
Oberdörster G, Elder A, Rinderknecht A. (2009). Nanoparticles and the Brain: Cause for Concern?J Nanosci Nanotechnol,Aug; 9(8): 4996-5007.
Kreyling WG, Semmler-Behnke M, Takenaka S, Moller W. (2013).Differences in the biokinetics of inhaled nano- versus micrometer-sized particlesAcc Chem Res,19; 46(3):714-22.
Graham UM, Yokel RA, Dozier AK, Drummy L, Mahalingam K, Tseng TM, Birch E and Fernback J. (2018). Analytical high-resolution electron microscopy reveals organ-specific nanoceria bioprocessing. Toxicologic Pathology 46 (1):47-61.
Information on Registered Substances comes from registration dossiers which have been assigned a registration number. The assignment of a registration number does however not guarantee that the information in the dossier is correct or that the dossier is compliant with Regulation (EC) No 1907/2006 (the REACH Regulation). This information has not been reviewed or verified by the Agency or any other authority. The content is subject to change without prior notice.
Reproduction or further distribution of this information may be subject to copyright protection. Use of the information without obtaining the permission from the owner(s) of the respective information might violate the rights of the owner.
