Registration Dossier
Registration Dossier
Data platform availability banner - registered substances factsheets
Please be aware that this old REACH registration data factsheet is no longer maintained; it remains frozen as of 19th May 2023.
The new ECHA CHEM database has been released by ECHA, and it now contains all REACH registration data. There are more details on the transition of ECHA's published data to ECHA CHEM here.
Diss Factsheets
Use of this information is subject to copyright laws and may require the permission of the owner of the information, as described in the ECHA Legal Notice.
EC number: 618-347-7 | CAS number: 9003-01-4
- Life Cycle description
- Uses advised against
- Endpoint summary
- Appearance / physical state / colour
- Melting point / freezing point
- Boiling point
- Density
- Particle size distribution (Granulometry)
- Vapour pressure
- Partition coefficient
- Water solubility
- Solubility in organic solvents / fat solubility
- Surface tension
- Flash point
- Auto flammability
- Flammability
- Explosiveness
- Oxidising properties
- Oxidation reduction potential
- Stability in organic solvents and identity of relevant degradation products
- Storage stability and reactivity towards container material
- Stability: thermal, sunlight, metals
- pH
- Dissociation constant
- Viscosity
- Additional physico-chemical information
- Additional physico-chemical properties of nanomaterials
- Nanomaterial agglomeration / aggregation
- Nanomaterial crystalline phase
- Nanomaterial crystallite and grain size
- Nanomaterial aspect ratio / shape
- Nanomaterial specific surface area
- Nanomaterial Zeta potential
- Nanomaterial surface chemistry
- Nanomaterial dustiness
- Nanomaterial porosity
- Nanomaterial pour density
- Nanomaterial photocatalytic activity
- Nanomaterial radical formation potential
- Nanomaterial catalytic activity
- Endpoint summary
- Stability
- Biodegradation
- Bioaccumulation
- Transport and distribution
- Environmental data
- Additional information on environmental fate and behaviour
- Ecotoxicological Summary
- Aquatic toxicity
- Endpoint summary
- Short-term toxicity to fish
- Long-term toxicity to fish
- Short-term toxicity to aquatic invertebrates
- Long-term toxicity to aquatic invertebrates
- Toxicity to aquatic algae and cyanobacteria
- Toxicity to aquatic plants other than algae
- Toxicity to microorganisms
- Endocrine disrupter testing in aquatic vertebrates – in vivo
- Toxicity to other aquatic organisms
- Sediment toxicity
- Terrestrial toxicity
- Biological effects monitoring
- Biotransformation and kinetics
- Additional ecotoxological information
- Toxicological Summary
- Toxicokinetics, metabolism and distribution
- Acute Toxicity
- Irritation / corrosion
- Sensitisation
- Repeated dose toxicity
- Genetic toxicity
- Carcinogenicity
- Toxicity to reproduction
- Specific investigations
- Exposure related observations in humans
- Toxic effects on livestock and pets
- Additional toxicological data
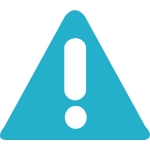
Endpoint summary
Administrative data
Link to relevant study record(s)
Description of key information
The toxicokinetic, metabolism and distribution data reported below, and in sections 7.1.1 Basic toxicokinetics and 7.1.2 Dermal absorption, apply to the analogue of the registered substance, acrylic acid.
Key value for chemical safety assessment
Additional information
Following oral administration of [14C]-Acrylic acid in rats and mice, a high percentage of the radiolabel (60 – 80 %) was rapidly absorbed and eliminated as 14CO2 within 24 hours by both species. Excretion in urine and faeces accounted for 1-4 %, respectively. In rats, about 19-25 % of the acrylic acid-derived radioactivity remained in the tissues examined after 72 hr, mostly in adipose tissue and muscle. High-performance liquid chromatography (HPLC) analysis of rat urine and rat and mouse tissues indicated that absorbed AA was rapidly metabolized by the ß-oxidation pathway of propionate catabolism. No unchanged AA was detected; however, several metabolites that were more polar than AA were measured, including 3-hydroxypropionate.
The presented results are consistent with the incorporation of AA into a secondary pathway for propionic acid metabolism in which 3 -hydroxypropionate is an intermediate. In this pathway, AA is first converted to acrylyl-CoA which is subsequently oxidized to 3 -hydroxypropionate. 3 -Hydroxypropionate is, in turn, metabolized to acetate and CO2 via malonic semialdehyde. The resultant acetate is then incorporated into intermediary metabolism. This pathway has been reported to be a major pathway for the metabolism of propionic acid in various insect and plant species, but is a secondary pathway in mammals.
On the other hand, reaction with reduced glutathion does not play a major role in the detoxification and metabolism of acrylic acid.
A hybrid CFD-PBPK inhalation model was constructed with the aim to evaluate the relationship between inhaled acrylic acid vapour concentration and the tissue concentration in various regions of the nasal cavity of rats and humans, respectively. The CFD-PBPK model simulations indicated that the olfactory epithelium of the human nasal cavity is exposed to two- to threefold lower tissue concentrations of a representative inhaled organic acid vapour, acrylic acid, than the olfactory epithelium of the rodent nasal cavity when the exposure conditions are the same. The magnitude of this difference varies somewhat with the specific exposure scenario that is simulated. The increased olfactory tissue dose in rats relative to humans may be attributed to the large rodent olfactory surface area (greater than 50% of the nasal cavity) and its highly susceptible location (particularly, a projection of olfactory epithelium extending anteriorly in the dorsal meatus region). In contrast, human olfactory epithelium occupies a much smaller surface area (less than 5% of the nasal cavity), and it is in a much less accessible dorsal posterior location. In addition, CFD simulations indicated that human olfactory epithelium is poorly ventilated relative to rodent olfactory epithelium. These studies suggest that the human olfactory epithelium is protected from irritating acidic vapours significantly better than rat olfactory epithelium due to substantive differences in nasal anatomy and nasal air flow.
Discussion on bioaccumulation potential result:
In Vivo Studies:
C3H mice and Fischer 344 rats, respectively, were treated by gavage (40 or 150 mg/kg bw) with [1-14C]-acrylic acid. Mice rapidly absorbed and metabolised orally administered acrylic acid, with about 80% of the dose exhaled as 14CO2 within 24 h. Excretion in urine and faeces accounted for approximately 3% and 1% of the dose, respectively. Elimination of the 14C radiolabel from plasma, liver and kidney was rapid but it was slower from fat. The disposition of orally administered acrylic acid in rats was similar to the results obtained from mice. High-performance liquid chromatography (HPLC) analysis of rat urine and rat and mouse tissues indicated that absorbed AA was rapidly metabolized by the ß-oxidation pathway of propionate catabolism. No unchanged AA was detected 1 h after oral administration; however, several metabolites that were more polar than AA were measured, including 3-hydroxypropionate. Neither AA nor its metabolites were detected at later times after oral administration (Black et al., 1995).
Sprague-Dawley rats received single oral doses of [2,3-14C]-acrylic acid (4, 40 or 400 mg/kg bw in a 0.5 % aqueous methylcellulose solution). Within 8 hours, 35-60% of the dose was eliminated from the animal, mostly as expired CO2. After 72 hours, 44-65% of the radioactivity had been eliminated via expired air, while 2.9-4.3% remained in urine, 2.4-3.6% in faeces and 18.9-24.6% in tissues examined (adipose tissue 9-15%, liver 1.7-2.2%, muscle 6.5-7.5% and blood 0.8-1.1%) (De Bethizy et al., 1987).
The HPLC profile of metabolites observed in the urine of rats indicated two major metabolites. One of the major metabolites co-eluted with 3-hydroxypropionic acid. Radioactivity could not be detected at the retention times corresponding to that of 2,3-epoxypropionic acid or N-acetyl-S-(2-carboxy-2-hydroxyethyl)cysteine leading to the conclusion that AA is not epoxidized to 2,3-epoxypropionic acid in vivo. This result was supported by an in vitro study. Hepatic microsomes were prepared using conventional methods from rats and incubations were started by the addition of 10 µL of [2,3-14C]-acrylic acid. No epoxidized metabolites could be detected and the parent compound was recovered from the incubation mixture unchanged (DeBethizy et al., 1987).
In addition, Glutathione Depletion Studies were conducted in rats that were administered doses of 4, 40, 400 or 1000 mg/kg bw AA by gavage. One hour following oral administration of acrylic acid in rats a significant depletion of NPSH in the glandular stomach was reported at doses above 4 mg/kg bw. In the forestomach NPSH depletion occurred at a dose of 1000 mg/kg bw. No significant effect of acrylic acid on NPSH in the blood or liver was observed (DeBethizy et al., 1987).
In Vitro Studies:
Dow Chemical (1979) have studied the metabolism of acrylic acid in rat tissue homogenates. Acrylic acid did not react with reduced glutathione either in the presence or absence of the soluble enzyme fraction. Non-protein sulfhydryl concentrations were not appreciably lower in blood after addition of acrylic acid in vitro (Dow Chemical, 1979; Miller et al., 1981).
The rate of 14CO2 formation from [14C]-acrylic acid was measured in vitro with preparations from rat liver hepatocytes. Rapid oxidation of acrylic acid to CO2 was observed. Mitochondria isolated from the liver homogenates were incubated with acrylic acid under the same conditions and yielded higher rates of acrylic acid-oxidation than homogenates. HPLC analysis of the mitochondrial incubation mixtures indicated 3-hydroxypropionic acid as a major metabolite of AA (Finch & Frederick, 1992).
Black et al. (1993) determined the rate of the in vitro oxidation of acrylic acid in 13 tissues of mice. The maximal rate of acrylic acid oxidation in kidney, liver and skin was 2890, 616 and 48 nmol/h/g, respectively. In remaining organs acrylic acid was oxidized at rates less than 40% of the rate in liver. 3-Hydroxypropionic acid was the only metabolite detected by HPLC analysis.
Acrylic acid oxidation rates and blood tissue partition coefficients were studied in slices of rat tissue using [1-14C]-acrylic acid. Acrylic acid oxidation in rat kidney and liver slices was described by saturable kinetics with maximal rates of about 4 and 2 μmol/h/g, respectively. Acrylic acid oxidation rates in 11 additional tissues were 40% or less than that in liver (Black & Finch, 1995).
Computational Modeling Data:
A hybrid computational fluid dynamics (CFD) and physiologically-based pharmacokinetic (PBPK) dosimetry inhalation model was constructed to estimate the regional tissue dose of acrylic acid in the rat and human nasal cavity, respectively (Frederick et al., 1998). This study provides a scientific basis for interspecies extrapolation of nasal olfactory irritants from rodents to humans. By using a series of short-term in vivo studies, in vitro studies with nasal explants, and computer modeling, regional nasal tissue dose estimates were made and comparisons of tissue doses between species were conducted. To make these comparisons, this study assumes that human and rodent olfactory epithelium have similar susceptibility to the cytotoxic effects of organic acids based on similar histological structure and common mode of action considerations. Interspecies differences in susceptibility to the toxic effects of acidic vapours are therefore assumed to be driven primarily by differences in nasal tissue concentrations that result from regional differences in nasal air flow patterns relative to the species-specific distribution of olfactory epithelium in the nasal cavity.
The rodent model uses two olfactory compartments to incorporate both the olfactory epithelium in the projection extending along the dorsal meatus and the ethmoid olfactory region. This model was based on a compartmental rat nasal model of Bush et al. (1998). The human model uses one olfactory compartment since the human nasal cavity lacks a counterpart for the rodent ethmoid olfactory region (Subramaniam et al., 1998). The liquid phase of the model of Bush et al. was modified to include the effect of buffering capacity on the ionization of the acid in the mucus, diffusion of both the ionized form of the acid and the non-ionized species, liquid:air partition coefficients, tissue:blood partition coefficients (Black and Finch, 1995), and metabolism of acrylic acid (Black and Finch, 1995).
A hybrid CFD-PBPK inhalation model was constructed with the aim to evaluate the relationship between inhaled acrylic acid vapour concentration and the tissue concentration in various regions of the nasal cavity of rats and humans, respectively. An explicit effort was made to derive the parameters for rat and human used in the model either from experimental data or from physicochemical principles without "fitting" model parameters (gas phase diffusivity: 0.1 cm2/sec; air minute volumes: 250 mL/min (rat), 7500 mL/min (human); blood flow to nasal cavity (human) estimated). Deposition of vapours in the rat nasal cavity is relatively insensitive to significant variation in the gas phase mass transport coefficients, but the human CFD-PBPK model was sensitive to variation in air phase and liquid phase parameters (liquid diffusivity, mucus:air partition coefficient).
Unidirectional simulations were conducted with the model at a flow rate of 500 mL/min (rat) to estimate the steady-state tissue concentration in the anterior olfactory epithelium lining the dorsal meatus of the rat nasal cavity over a wide range of acrylic acid vapour concentrations (0 to 25 ppm for one hour). A dose-response of acrylic acid exposures was simulated for an adult resting male rat and an adult resting male human using the appropriate inspiratory flow rate (based on the minute volumes of each species), nasal anatomy, and nasal air flow patterns from CFD simulations. The cyclic flow simulation was conducted for a reference resting rat and human exposed to 2 ppm acrylic acid for 3 min (minute volume 250 mL/min (rat), 7500 mL/min (human)).
The acute inhalation, and in vitro studies have demonstrated that the nasal olfactory epithelium is the most sensitive tissue to the effects of inhalation exposure to organic acids and that the sustentacular cells are the most sensitive cell type of this epithelium. The CFD-PBPK model simulations indicated that the olfactory epithelium of the human nasal cavity is exposed to two- to threefold lower tissue concentrations of a representative inhaled organic acid vapour, acrylic acid, than the olfactory epithelium of the rodent nasal cavity when the exposure conditions are the same. The magnitude of this difference varies somewhat with the specific exposure scenario that is simulated. The increased olfactory tissue dose in rats relative to humans may be attributed to the large rodent olfactory surface area (greater than 50% of the nasal cavity) and its highly susceptible location (particularly, a projection of olfactory epithelium extending anteriorly in the dorsal meatus region). In contrast, human olfactory epithelium occupies a much smaller surface area (less than 5% of the nasal cavity), and it is in a much less accessible dorsal posterior location. In addition, CFD simulations indicated that human olfactory epithelium is poorly ventilated relative to rodent olfactory epithelium. These studies suggest that the human olfactory epithelium is protected from irritating acidic vapours significantly better than rat olfactory epithelium due to substantive differences in nasal anatomy and nasal air flow.
Discussion on absorption rate:
The absorption of [14C]-acrylic acid from acetone, water, and phosphate buffer was measured through human and mouse skin in vitro. Membranes were mounted in glass diffusion cells and acrylic acid was applied in each solvent at 0.01 %, 0.1 %, 1 %, and 4 %, respectively (100 µL/cm2) under occlusive conditions. Samples were taken from the receptor solutions at recorded times, between 0 and 32 hr, and assayed for 14C content which was regarded as equivalent to acrylic acid. Steady state absorption rates were calculated to be between 0.007 µg/cm2/hr (human, 0.01 % AA in phosphate buffer) and 201 µg/cm2/hr (mouse, 4 % AA in acetone). Thus, absorption rates were influenced by the vehicle (acetone > water > phosphate buffer) and were proportional to the applied concentration in each vehicle. Mouse skin was 3 times more permeable than human skin under the conditions of this in vitro study (BAMM 1988).
C3H mice and Fischer 344 rats, respectively, were treated dermally (10 or 40 mg/kg bw in acetone) with [1-14C]-acrylic acid. After cutaneous application to mice, about 12% of the dose was absorbed, while the remainder was apparently evaporated. Approximately 80% of the absorbed fraction of the dose was metabolised to 14CO2within 24 h. Excretion in urine and faeces each accounted for less than 0.5% of the dose. Elimination of radioactivity from plasma, liver, and kidney was rapid; however, levels in fat were higher at 72 h (0.5% of the higher dose) than at 8 h (0.1% of the higher dose). After cutaneous administration to rats, 19-26% of the dose was absorbed. Disposition of the absorbed fraction of the dose was similar to results found in mice. Results from an in vitro experiment with rat skin (Frantz cell) showed that at least 60 % of the applied dose evaporated and about 25% was absorbed, confirming the in vivo results. High-performance liquid chromatography (HPLC) analysis of rat urine and rat and mouse tissues indicated that absorbed AA was rapidly metabolized by the ß-oxidation pathway of propionate catabolism (Black et al., 1995).
Information on Registered Substances comes from registration dossiers which have been assigned a registration number. The assignment of a registration number does however not guarantee that the information in the dossier is correct or that the dossier is compliant with Regulation (EC) No 1907/2006 (the REACH Regulation). This information has not been reviewed or verified by the Agency or any other authority. The content is subject to change without prior notice.
Reproduction or further distribution of this information may be subject to copyright protection. Use of the information without obtaining the permission from the owner(s) of the respective information might violate the rights of the owner.
