Registration Dossier
Registration Dossier
Data platform availability banner - registered substances factsheets
Please be aware that this old REACH registration data factsheet is no longer maintained; it remains frozen as of 19th May 2023.
The new ECHA CHEM database has been released by ECHA, and it now contains all REACH registration data. There are more details on the transition of ECHA's published data to ECHA CHEM here.
Diss Factsheets
Use of this information is subject to copyright laws and may require the permission of the owner of the information, as described in the ECHA Legal Notice.
EC number: 203-542-8 | CAS number: 108-01-0
- Life Cycle description
- Uses advised against
- Endpoint summary
- Appearance / physical state / colour
- Melting point / freezing point
- Boiling point
- Density
- Particle size distribution (Granulometry)
- Vapour pressure
- Partition coefficient
- Water solubility
- Solubility in organic solvents / fat solubility
- Surface tension
- Flash point
- Auto flammability
- Flammability
- Explosiveness
- Oxidising properties
- Oxidation reduction potential
- Stability in organic solvents and identity of relevant degradation products
- Storage stability and reactivity towards container material
- Stability: thermal, sunlight, metals
- pH
- Dissociation constant
- Viscosity
- Additional physico-chemical information
- Additional physico-chemical properties of nanomaterials
- Nanomaterial agglomeration / aggregation
- Nanomaterial crystalline phase
- Nanomaterial crystallite and grain size
- Nanomaterial aspect ratio / shape
- Nanomaterial specific surface area
- Nanomaterial Zeta potential
- Nanomaterial surface chemistry
- Nanomaterial dustiness
- Nanomaterial porosity
- Nanomaterial pour density
- Nanomaterial photocatalytic activity
- Nanomaterial radical formation potential
- Nanomaterial catalytic activity
- Endpoint summary
- Stability
- Biodegradation
- Bioaccumulation
- Transport and distribution
- Environmental data
- Additional information on environmental fate and behaviour
- Ecotoxicological Summary
- Aquatic toxicity
- Endpoint summary
- Short-term toxicity to fish
- Long-term toxicity to fish
- Short-term toxicity to aquatic invertebrates
- Long-term toxicity to aquatic invertebrates
- Toxicity to aquatic algae and cyanobacteria
- Toxicity to aquatic plants other than algae
- Toxicity to microorganisms
- Endocrine disrupter testing in aquatic vertebrates – in vivo
- Toxicity to other aquatic organisms
- Sediment toxicity
- Terrestrial toxicity
- Biological effects monitoring
- Biotransformation and kinetics
- Additional ecotoxological information
- Toxicological Summary
- Toxicokinetics, metabolism and distribution
- Acute Toxicity
- Irritation / corrosion
- Sensitisation
- Repeated dose toxicity
- Genetic toxicity
- Carcinogenicity
- Toxicity to reproduction
- Specific investigations
- Exposure related observations in humans
- Toxic effects on livestock and pets
- Additional toxicological data
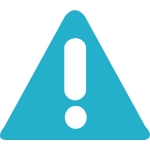
Endpoint summary
Administrative data
Link to relevant study record(s)
- Endpoint:
- basic toxicokinetics in vivo
- Type of information:
- experimental study
- Adequacy of study:
- key study
- Reliability:
- 2 (reliable with restrictions)
- Rationale for reliability incl. deficiencies:
- other: acceptable well-documented publication, which meets basic scientific principles
- Objective of study:
- distribution
- Principles of method if other than guideline:
- DMAE - more precisely that p-acetamidobenzoate salt of deanol - was administered i.p. to rats or mice and the Acetylcholine (ACh) levels in the brain or different brain sections were determined via a gaschromatigraphic method.
- GLP compliance:
- not specified
- Species:
- rat
- Strain:
- Sprague-Dawley
- Sex:
- male
- Details on test animals or test system and environmental conditions:
- Sprague-Dawley rats (male, 180-200 g) were obtained from Zivic-Miller Laboratories (Allison Park, Pa.).
Either Swiss-Webster mice from Hilltop Lab Animals, Inc. (Scottdale, Pa.) or Charles River mice from Charles River Laboratories, Inc. (Wilmington, Mass.) were used (either sex, 25-30 g) as specified in the text. - Route of administration:
- intraperitoneal
- Vehicle:
- not specified
- Details on exposure:
- Route: injection
- Duration and frequency of treatment / exposure:
- one pretreatment via i.p. injection
- Dose / conc.:
- 33.3 mg/kg bw/day
- Remarks:
- rats
- Dose / conc.:
- 100 mg/kg bw/day
- Remarks:
- rats
- Dose / conc.:
- 300 mg/kg bw/day
- Remarks:
- rats / mice
- Dose / conc.:
- 900 mg/kg bw/day
- Remarks:
- rats / mice
- Dose / conc.:
- 3 000 mg/kg bw/day
- Remarks:
- mice
- No. of animals per sex per dose / concentration:
- 5 - 10 depending on the experiments
- Control animals:
- yes
- Positive control reference chemical:
- no data
- Details on study design:
- The concentrations of deanol, ACh and Ch were determined in mice or rats treated acutely with deanol (i.p.) or saline (an equivalent volume on a per weight basis; i.p.)
Brain dose-concentration studies. Whole brain levels of deanol, ACh and Ch were determined in female Swiss-Webster mice after pretreatment 30 minutes earlier with 33.3, 100, 300 or 900 mg/kg of deanol. A similar study was conducted using male Swiss-Webster mice pretreated 30 minutes earlier with 300, 900 or 3000 mg/kg of deanol.
Regional brain studies.
1) Male Swiss-Webster mice were pretreated with either 300 or 900 mg/kg of deanol 30 minutes prior to killing. Deanol and ACh concentrations were determined in whole brains from half of the treated group and in both striate from mice in the other half of the treated group.
2) Male rats were pretreated with 550 mg/kg of deanol and killed 15 minutes later. Concentrations of deanol and ACh were measured in whole brain as well as in the cortex, striatum and hippocampus from rats receiving identical treatment.
Brain time-course study. Swiss-Webster mice (either sex) were pretreated with 300 mg/kg of deanol 1, 2, 6, 8, 12 or 30 minutes before sacrifice with microwave irradiation. Control mice were sacrificed at identical intervals after receiving saline injections and also included a noninjected group reported as zero minutes. Whole brain levels of deanol, ACh and Ch were determined.
Plasma time-course study. Swiss-Webster or Charles River male mice were pretreated with 300 mg/kg of deanol 1, 2, 6, 8, 12, 15, 20 or 32 minutes before decapitation. A group of noninjected mice were included in the control group and, thus, constituted a zero minute pretreatment group. Only deanol levels were determined in the plasma of the treated and control mice. - Details on dosing and sampling:
- The brain was removed from the skull, dissected when necessary at room temperature, weighed and immediately homogenized in ice-cold 0.4 N perchloric acid for 20 seconds.
In order to assay for deanol, ACh and Ch in the same tissue, each resulting supernatant was split into two separate samples. One sample (0.8 mL) was assayed for deanol, whereas the other sample (2.0 mL) was assayed for ACh and Ch plus deanol
Pooled brain supernatant (pH 4.2-4.4) from noninjected animals was used for the external standard samples to which known concentrations of deanol and preanol or ACh, Ch and PCh were added.
Buffer blank samples containing 2.5 mL ofpH 4 ammonium acetate buffer, instead of tissue supernatant, were also routinely included.
Brains from mice and rats killed by microwave irradiation were assayed for free deanol concentration. Also brains and plasma from mice killed by decapitation were analyzed for basal deanol levels. Peripheral tissues-liver, lung and heart-were taken from rats which had been killed by microwave irradiation; however, the peripheral tissues were not irradiated in these animals. These tissues were also assayed for deanol content.
1 mL heparinized blood was taken from the rats - Statistics:
- two-tailed Student’s t test
- Preliminary studies:
- no data
- Details on absorption:
- rapid distribution after i.p. administration
- Details on distribution in tissues:
- With increasing concentration of deanol administered, the percentage of the total amount of the drug recovered in the brain increased but never exceeded 0.2 % of the total administered dose. Even at the highest injected concentration, 3000 mg/kg, which approximated the LD50 in the mice, less than 0.2 % of the administered dose was found in the brain after the 30-minute pretreatment period. No sex difference in the response to deanol was observed.
The whole brain ACh levels of the animals treated with deanol over the entire dosage range did not differ significantly from control levels. The female mice did have a lower basal whole brain ACh level than that of the male mice.
Ch levels were also not significantly different from control levels in either group for any of the doses of deanol checked.
The regional distribution study in rats: whole brain, cortex, striatum and hippocampus
The deanol level in whole brain appears to reach a peak between 16 to 20 minutes after intraperitoneal injection of deanol.
From the plasma level curve for deanol, it is evident that deanol rapidly entered the systemic circulation after i.p. injection. - Details on excretion:
- Assuming plasma deanol levels decay exponentially, an estimation of the plasma half-life for deanol from this study in the mouse is 15 minutes. There may be, however, a two-phase decline in plasma deanol levels with an early phase having a half-life <10 minutes and a later phase >15 minutes.
- Metabolites identified:
- not specified
- Details on metabolites:
- no data
- Conclusions:
- Interpretation of results: no bioaccumulation potential based on study results
No free endogenous deanol was detected in either whole brains or brain areas (cortex, striatum and hippocampus) from microwaved mice or rats. Whole brains removed from decapitated mice also contained no free deanol greater than 300 pmol (= 0.3 nmol). Even when the tissue extract assayed was made 3 times more concentrated than usual, no free deanol was found. Also, no endogenous deanol was detectable in the peripheral tissues analyzed. Plasma taken from untreated mice did not contain any free deanol. - Executive summary:
Zahniser and colleagues investigated in 1977, the role of DMEA as a possible precursor of acetylcholine. In their test, they could not prove that free deanol exists in the tissues examined in the unbound form or its concentration is below the sensitivity of the gas chromatographic assay used. Endogenous level of free deanol in the whole brain less than 1 nmol/g of tissue (wet weight). No significant elevation in mouse whole brain ACh levels after i.p. administration of DMAE could be found. Additionally there was no postmortem rise in the concentration of free deanol in the whole mouse brain. In other words, no deanol could be detected in any of the samples. On the other hand, free Ch levels rose and levels of ACh dropped significantly from control levels in brains left for 5 or 15 minutes at room temperature. Deanol, therefore, seems to be relatively nontoxic because of its high incorporation into endogenous lipid-forming pathways and its poor penetration into the central nervous system.
The primary source for de novo synthesis of Ch in the mammal is, in fact, the liver where phosphatidyldimethylaminoethanol has been shown to be the immediate precursor of phosphatidylcholine. Furthermore, evidence exists that exogenously administered deanol can also serve as a precursor of phosphoryl and phosphatidylcholine in the liver.
In conclusion, DMAE ist not likely to be a direct precursor of brain ACh in rodents. The only increase in ACh levels detected after acute deanol administration occurred selectively in mouse striatum following the massive dose of 900 mg/kg of this agent.
Reference
Only the cation exchange resins Amberlite CG-120 (Stavinoha and Weintraub, 1974) and Dowex 50W-X8 consistently extracted greater than 80% of all three labeled tracers from the tissue samples. The two liquid extraction procedures extracted only 20% of the deanol, whereas the precipitation procedure extracted 50%. Consequently it was decided to use Dowex 50W-X8 resin in subsequent experiments.
The limit of sensitivity of this deanol assay was determined to be 300 pmol (picomol).
Description of key information
Summary of available literature
Key value for chemical safety assessment
- Bioaccumulation potential:
- no bioaccumulation potential
- Absorption rate - oral (%):
- 100
- Absorption rate - dermal (%):
- 100
- Absorption rate - inhalation (%):
- 100
Additional information
The available data depicted below is generated mainly with structurally similar analogues of DMAE. However, the results of these studies can be used for dimethylaminoethanol due to the following reasons.
The target substance dimethylaminoethanol and either the source substance the dimethylaminoethanol hydrochloride or p-acetamidobenzoate salt of dimethylaminoethanol belong to the group of tertiary aliphatic amines. The solvation of either, dimethylaminoethanol and dimethylaminoethanol hydrochloride and dimethylaminoethanol and the p-acetamidobenzoate salt of dimethylaminoethanol in water results in solutions of the dimethylaminoethanol cation (common "breakdown product"). The respective anions are not expected to bear a relevant intrinsic property, allowing one in general to focus on the dimethylammonium cation. The dimethylaminoethanol cation is believed to act and to be metabolised by the same mechanisms by microorganisms and by other classes of living organisms.
Therefore all source substances are expected to follow the same toxicokinetic pattern.
Absorption, distribution and excretion
DMAE is absorbed (either from the small intestine after oral dosing or from the bloodstream after injections), and rapidly transported to the liver where much of it is metabolized (Zahniser et al., 1977). Approximately 280 nmol (25.2 μg) DMAE/gram plasma was observed in male mice about ten minutes after receiving 300 mg (3.30 mmol) DMAE/kg, intraperitoneally (Zahniser et al., 1977).
The absorption of cyprodenate maleate , the cyclohexylpropionic acid ester of DMAE and DMAE (administered as its hydrochloride salt) were investigated in pigs and rats (Dormard et al., 1975a). Cyprodenate maleate was totally absorbed well from the digestive tract and distributed to tissues and organs in both species. Approximately 2.41, 1.30, and 0.20 % of an administered dose of 30 mg/kg (0.13 mmol/kg) (with 100 μCi) of 14C-cyprodenate was found in the liver, brain and plasma, respectively, five minutes after intravenous dosing in male rats. The two labeled products were primarily excreted in the urine (30 -35 % of the dose in 72h in rats and 6 % of the dose in 48 h pigs) following oral administration of cyprodenate. Radioactivity found in feces was practically nil and in rats the biliary elimination of the drug was very weak. Radioactivity expired as 14CO2 was negligible (around 1 % of the administered dose in 8h) however, this value increases as a function of time, becoming 4 % in 24h. In rats, the maximum radioactivity was found at 45 min to 1h after oral administration of 14C-cyprodenate. These values decreased slowly until 3h when they begin to increase again. The rising of blood level values is practically the same for pigs, the maximum being attained at 1h. Two labeled products bound to the plasma proteins in both species.
Metabolism of DMAE
Dormard and colleagues investigated the metabolites of 14C-cyprodenate in rats and pigs and 14C-DMAE (administered as its hydrochloride salt) in rat's blood, bile, urine, and organs such as the brain and the liver. They could prove that 14C-cyprodenate is a precursor of DMAE, (rapid hydrolysation to DMAE and cyclohexyl propionic acid). The metabolite DMAE is the major metabolite of cyprodenate. DMAE is then oxidized very rapidly and the maximum value of DMAE-N-oxide is found 30 min after medication. This metabolite is the only one to be eliminated in the urine and considering the small quantity of excreted radioactivity (30 % of the administered dose in 24 h) it would be most likely that this metabolic pathway (N-oxidation) corresponds to a liver detoxification of the product. This detoxification competes with the incorporation of DMAE in the biosynthesis of phopholipids. There is also a reversible reaction between DMAE and its N-oxide; there is therefore an incorporation of DMAE in the metabolic cycle of phopholipids depending on the regeneration of N-oxide. At the level of the liver and brain, identification of 14C-labeled phosphoryl DMAE (P-DMAE) and glycerophosphatidyl DMAE (GP-DMAE) suggests that for the latter there is a biosynthesis similar to that of cephalins and lecithins, the 14C-DMAE being rapidly phophorylated as is found at liver and brain levels of P-DMAE at 5 min. The fact that 14C-GP-choline is found in the plasma and brain 8 -24 h and in the liver 3 -24 h after medication seems to prove that 14C-GP-DMAE is methylated to give GP-choline, which is transformed to choline, the latter being identified in the brain at 24 h. The fact that the unidentified metabolite produces 14C-choline by hydrolysis supports the hypothesis that it is cytidinediphosphocholine (CDP-choline). Furthermore, because of the labeled position of 1 -14C-DMAE during the reversible reaction between serine and glycine, 14CO2 must be produced during methyl radicals' formation on the one hand, and glyoxylic catabolism on the other.
In fetal rat brain aggregating cell cultures exposed to varying concentrations of [3H]DMAE for 72 hours, 95 %, and 5 % of the radioactivity was associated with phosphatidyl-DMAE and phosphatidylcholine, respectively. The rate of formation of radioactive products was concentration dependant up to 4 mM [3H]DMAE, the highest concentration tested. The authors calculated an apparent half-life of 24 hours (Dainous and Kanfer, 1988).
Penetration through the blood-brain barrier
Concerning the distribution of DMAE, it was shown that DMAE can cross the blood brain barrier to a certain extent after intraperitoneal administration to rats or mice (Zahniser et al., 1977). This was also proven by Dormard et al. where pigs and rats were dosed orally with cyprodenate maleate, the cyclohexylpropionic acid of DMAE or DMAE administered as its hydrochloride salt: animals sacrificed 1 h after receiving the labeled product proved that DMAE (14C-DMAE) hardly crosses the blood-brain barrier. Cyprodenate, a psychotic drug, crosses the hemo-cyphalic barrier more easily than DMAE and this procedure is achieved without modification of the permeability and can be explained by the liposolubility of the molecule after loss of maleic acid (Dormard et al., 1975a).
Differential penetration of the blood-brain barrier by several DMAE derivatives has been noted. Miyazaki et al. (1976) found that radiolabeled DMAE p-chlorophenoxyacetate was found in higher concentrations in the brain than radiolabeled DMAE after intravenous treatment of mice. Higher levels of DMAE were found in the brain after dosing with centrophenoxine than with DMAE, possibly due to improved penetration of the blood-brain barrier by the esterified form of DMAE (South, undated). Similarly, Dormard et al. (1975a) reported that, relative to radiolabeled DMAE, radiolabeled cyprodenate maleate was more rapidly absorbed and accumulated to a large extent in the brain.
DMAE as a precursor either choline or acetylcholine
As it is speculated, that dimethylaminoethanol (DMAE, or deanol) is a possible precursor of acetylcholine, this issue was investigated by Zahniser and coworkers (Zahniser et al., 1977). No prove could be given that DMAE is a precursor of acetylcholine in brain, since after acute DMAE administration to rodents the level of whole brain acetylcholine did not raise significantly. Endogenous level of free deanol in the whole brain was less than 1 nmol/g of tissue (wet weight). They did not find free DMAE in the tissues in the unbound form. Additionally there was no postmortem rise in the concentration of free deanol in the whole mouse brain.
There is data presented in the "Review of Toxicological Literature" (2002).
Several enzymatic studies have been performed to demonstrate the presence of choline acetyltransferase or phosphatidylethanolamine N-methyltransferase in an effort to identify acetylcholine or choline in body tissues. Bishop et al. (1976) identified DMAE acetate in both bull and human spermatozoa. DMAE propionate was only identified in bull spermatozoa. Both acetylcholine and choline acetyltransferase were found in bull and human spermatozoa samples, suggesting in situ synthesis of acetylcholine.
Yang et al. (1988) used the existence of phosphatidylethanolamine N-methyltransferase in rat mammary tissue as supporting evidence for de novo synthesis of choline in breast tissue. Their results suggested that at least two distinct enzyme activities were involved, one catalyzing the methylation of phosphatidylethanolamine to phosphatidyl-N-methylethanolamine and the other catalyzing the further methylation of phosphatidyl-N-methylethanolamine to form phosphatidyl-N,N-dimethylethanolamine. When exogenous phosphatidyl-DMAE was added to homogenates of rat mammary tissue, twice as much phosphatidylcholine was produced from lactating rats as from non-lactating rat tissue. Similar activities were identified in homogenates of mammary tissue from non-lactating humans, supporting the hypothesis that some choline found in breast milk could be synthesized de novo in the mammary gland.
The most significant results reported in Jope and Jenden (1979) as a result of DMAE treatment was an increase in the concentration of choline in both the plasma and the brain of treated rats, although the mechanism for this phenomenon was unknown. Since it was known that DMAE inhibits the influx of choline to the brain across the blood brain barrier it was possible that DMAE also inhibited the efflux of choline from the brain, resulting in an accumulation in the brain. It was unclear to what extent DMAE is methylated and substituted into acetylcholine. Early reports indicated that the DMAE that crossed the blood-brain barrier was methylated to form choline and then incorporated into acetylcholine (Goldberg and Silbergeld, 1974; Haubrich et al., 1975; both cited by Jope and Jenden, 1979; De Silva, 1977; Millington et al., 1978; cited by HSDB, 1996). Other studies fail to support this pathway (Ansell and Spanner, 1975; Freeman and Jenden, 1976; cited by Jope and Jenden, 1979, Zahniser et al., 1977). Jope and Jenden confirmed previous reports, through both in vivo and in vitro studies, that DMAE was not methylated to form choline in brain tissues. Neither acute (in vitro) nor chronic (in vivo) treatments with [2H6]DMAE had the capacity to alter levels of acetylcholine in the brain tissues. Treatment with HC-3, effectively reducing the concentration of acetylcholine in the brain, did not promote acetylcholine synthesis from DMAE in the rat brain. Although DMAE uptake, which is diffusion-dependant, was unaffected by HC-3, acetylcholine levels dropped dramatically, failing to support the contention that DMAE can supplement the synthesis of acetylcholine. Zahniser et al. concluded that the presence of N-methylaminoethanol or DMAE, both precursors in the biosynthesis of choline, did not stimulate the endogenous synthesis of either choline or acetylcholine in adult rats maintained on choline-deficient diets (Zahniser, 1978). Other authors hypothesized that DMAE is not methylated in brains but in other organs in rodents. In a study using DMAE p-chlorophenoxyacetic acid, Wood and Peloquin (1982) found that treatment of rats (dose and route not provided) resulted in increase levels of choline in the central nervous system of rats. These increased levels were accompanied by elevated steady state levels for acetylcholine within the hippocampus. DMAE was only about half as effective as DMAE p-chlorophenoxyacetate in increasing levels of choline in rat brains. The observed changes in choline levels were primarily extraneuronal. No consistent alterations in acetylcholine turnover were measured. Pedata et al. (1977) reported that DMAE (Deaner) did not affect acetylcholine brain concentrations in rats, cause behavioral changes, or antagonize the effect of HC-3 [2,2´-(4,4´-biphenylene)bis(2-hydroxy-4,4- dimethylmorpholinium)], an inhibitor of high-affinity transport of deuterated choline into cholinergic nerve endings, on striatal acetylcholine. Danysz et al. (1967b), on the other hand, reported a dose-dependant increase in acetylcholine levels in the brains, with peaks at 11 (higher doses) and 14 days (lower doses) of mice (strain and sex not provided) dosed with DMAE (175 to 350 mg/kg; 1.95 to 3.90 mmol/kg).
Conclusion:
DMAE is well absorbed via digestive tract and rapidly transported to the liver where much of it is metabolized. DMAE is oxidized to N-oxide, representing the primary urinary metabolite. However, the excretion either unchanged DMAE or oxidized DMAE does not compile the administered dose. There are contradictive results regarding the fate of the remaining DMAE. On the one hand, it is suggested that DMAE is methylated to choline and incorporated into phospholipids or crossed blood-brain barrier, is methylated to choline and then to acetylcholine. On the other hand, there are assumptions that DMAE is demethylated to ethanolamine and then routed toward normal metabolic pathway.
Information on Registered Substances comes from registration dossiers which have been assigned a registration number. The assignment of a registration number does however not guarantee that the information in the dossier is correct or that the dossier is compliant with Regulation (EC) No 1907/2006 (the REACH Regulation). This information has not been reviewed or verified by the Agency or any other authority. The content is subject to change without prior notice.
Reproduction or further distribution of this information may be subject to copyright protection. Use of the information without obtaining the permission from the owner(s) of the respective information might violate the rights of the owner.
