Registration Dossier
Registration Dossier
Data platform availability banner - registered substances factsheets
Please be aware that this old REACH registration data factsheet is no longer maintained; it remains frozen as of 19th May 2023.
The new ECHA CHEM database has been released by ECHA, and it now contains all REACH registration data. There are more details on the transition of ECHA's published data to ECHA CHEM here.
Diss Factsheets
Use of this information is subject to copyright laws and may require the permission of the owner of the information, as described in the ECHA Legal Notice.
EC number: 271-384-7 | CAS number: 68553-00-4 A distillate oil having a minimum viscosity of 900 SUS at 37.7°C (100°F) to a maximum of 9000 SUS at 37.7°C (100°F).
- Life Cycle description
- Uses advised against
- Endpoint summary
- Appearance / physical state / colour
- Melting point / freezing point
- Boiling point
- Density
- Particle size distribution (Granulometry)
- Vapour pressure
- Partition coefficient
- Water solubility
- Solubility in organic solvents / fat solubility
- Surface tension
- Flash point
- Auto flammability
- Flammability
- Explosiveness
- Oxidising properties
- Oxidation reduction potential
- Stability in organic solvents and identity of relevant degradation products
- Storage stability and reactivity towards container material
- Stability: thermal, sunlight, metals
- pH
- Dissociation constant
- Viscosity
- Additional physico-chemical information
- Additional physico-chemical properties of nanomaterials
- Nanomaterial agglomeration / aggregation
- Nanomaterial crystalline phase
- Nanomaterial crystallite and grain size
- Nanomaterial aspect ratio / shape
- Nanomaterial specific surface area
- Nanomaterial Zeta potential
- Nanomaterial surface chemistry
- Nanomaterial dustiness
- Nanomaterial porosity
- Nanomaterial pour density
- Nanomaterial photocatalytic activity
- Nanomaterial radical formation potential
- Nanomaterial catalytic activity
- Endpoint summary
- Stability
- Biodegradation
- Bioaccumulation
- Transport and distribution
- Environmental data
- Additional information on environmental fate and behaviour
- Ecotoxicological Summary
- Aquatic toxicity
- Endpoint summary
- Short-term toxicity to fish
- Long-term toxicity to fish
- Short-term toxicity to aquatic invertebrates
- Long-term toxicity to aquatic invertebrates
- Toxicity to aquatic algae and cyanobacteria
- Toxicity to aquatic plants other than algae
- Toxicity to microorganisms
- Endocrine disrupter testing in aquatic vertebrates – in vivo
- Toxicity to other aquatic organisms
- Sediment toxicity
- Terrestrial toxicity
- Biological effects monitoring
- Biotransformation and kinetics
- Additional ecotoxological information
- Toxicological Summary
- Toxicokinetics, metabolism and distribution
- Acute Toxicity
- Irritation / corrosion
- Sensitisation
- Repeated dose toxicity
- Genetic toxicity
- Carcinogenicity
- Toxicity to reproduction
- Specific investigations
- Exposure related observations in humans
- Toxic effects on livestock and pets
- Additional toxicological data
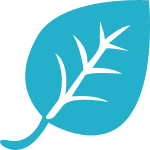
Endpoint summary
Administrative data
Description of key information
Additional information
To quantify environmental exposure resulting from multimedia distribution and degradation of hydrocarbon components that comprise a complex petroleum substance the ‘Hydrocarbon Block Method’, has been proposed by CONCAWE (1996) and EC (2003) and subsequently implemented in REACH (ECHA, 2008). In this approach, individual hydrocarbons with different partitioning and degradation properties are used to simulate petroleum substance fate in the environment.
Hydrocarbons are degraded (under aerobic conditions) via mono-oxygenases or di-oxygenases, and are subsequently carboxylated and ultimately hydroxylated. In further assessing the type of metabolites formed, it has been demonstrated that for all the major classes of hydrocarbons, the major metabolites are in most cases less toxic, and always less bioaccumulative than the parent molecule. Consequently, it is concluded that for PBT and risk assessment purposes, the metabolites of hydrocarbons do not require any further assessment. This is further supported by CONCAWE 2019 (An Evaluation of the Persistence, Bioaccumulation and Toxicityof Petroleum Hydrocarbons) attached to IUCLID section 13.
Specific hydrocarbon classes and how they degrade
1. Alkanes
The primary route of degradation for alkanes is to the alcohol. For hydrocarbons with longer chain lengths, it is probable that the occurrence of formation of the secondary alcohol increases, (Kachholz and Rehm , 1977). Fritsche and Hofrichter (2000) make similar observations.
2. Isoalkanes
The major difference between the metabolism under aerobic conditions of alkanes and isoalkanes relates to the impact of the branching on the rate of metabolism (Fritsche and Hofrichter, 2000). However, the initial metabolites are always alcohols.
3. Naphthenics (Cycloalkanes)
Naphthenics (or cycloalkanes) are relatively resistant to microbial attack as the absence of an exposed terminal methyl group hinders the primary attack (Fritsche and Hofrichter, 2000). The route of degradation of cycloalkanes is again generally via the alcohol and as a general rule alkyl side chains on cycloalkanes facilitate degradation.
4. Aromatic compounds
Given that a large number of aromatic compounds are formed by organisms, for example, aromatic amino acids, phenols, or quinines, it is not surprising that many microorganisms have evolved catabolic pathways to degrade them. Hence aromatic hydrocarbon molecules are readily degraded by microorganisms when the respective molecules are similar to other natural compounds. They are converted enzymatically to the natural intermediates of degradation, for example catechol and protecatechuate (Fritsche and Hofrichter, 2000). Although the introduction of aliphatic substituents normally alters the location in the molecule where the initial microbial attack takes place, the eventual degradation products should not be significantly different.
5. Polyaromatic compounds
The biochemical pathway for the aerobic biodegradation of PAHs has been extensively investigated. It is understood that the initial step in the aerobic catabolism of a PAH molecule by bacteria uses a multicomponent enzyme system to oxidise the PAH to a dihydrodiol. These dihydroxylated intermediates may then be processed through either an ortho cleavage type of pathway, in which ring fission occurs between the two hydroxylated carbon atoms, or a meta- cleavage type of pathway, which involves cleavage of the bond adjacent to the hydroxyl groups, leading to central intermediates such as protocatechates and catechols. These compounds are further converted to tricarboxylic acid cycle intermediates (van der Meer et al. 1992). For the lower molecular weight PAHs, the most common route involves the fission into a C3 compound and a hydroxyl aromatic acid compound. The aromatic ring can thereafter either undergo direct fission or can be subjected to decarboxylation, leading to the formation of a dihydroxylated compound. This compound can be dissimilated as described above. When degraded via these pathways, the low molecular weight PAHs can be completely mineralized to CO2 and H2O (Volkering and Breure, 2003).
The following observations were made by Volkering and Breure (2003);
- Many bacterial and fungal species have the enzymatic capacity to oxidise PAHs
- The aerobic transformation of PAHs always involve incorporation of oxygen into the molecule
- The initial step is usually performed via a dioxygenase and forms a dihydrodiol
Their conclusions were that the transformation products of PAHs were in general more polar than the parent and that there were metabolites that were potentially harmful to mammals and could also have important environmental consequences.
Photodegradation via photolysis is a major source of elimination of PAHs from various environmental compartments (Nikolaou et al. 1984), however, this is not the only pathway that occurs. For instance, anthracene is able to form the photoproduct 9,10-anthraquinone (ATQ) (Fox and Olive 1979; Nikolaou et al. 1984; Mallakin et al. 2000). There are a wide variety of other oxyPAHs that are formed via PAH photomodification. Some of these include phenanthrenequinone (McConkey et al. 1997), many hydroxylated anthraquinones (Mallakin et al. 2000; Brack et al. 2003), and a host of other unidentified compounds, resulting from the photomodification of a variety of PAHs (Huang et al. 1997).
There is little specific data available to evaluate the persistence and bioaccumulation of specific PAH photodegradation products. However, accompanying photomodification, which is generally via oxygenation, should lead to an increase in susceptibility to biotransformation in a similar manner to that observed after phase I metabolism by the mixed function oxidases (Prince and Walters, 2006) which will minimise the risk of persistence and bioaccumulation of such compounds.Degradation in the environment is a result of abiotic processes and biodegradation. The relative importance of these processes will depend upon the environmental compartment to which the individual components of the petroleum product partition. In general, abiotic processes are important in the atmosphere, whilst biodegradation is the principle mechanism of the breakdown of lower carbon chain length products in water and soil. Direct photolysis is not expected to be a major degradation pathway for many of the hydrocarbon components in petroleum substances and neither is hydrolysis, as the components of petroleum products lack hydrolysable functional groups.
The combined role of partitioning and degradation properties of constituent hydrocarbons on environmental fate and resulting exposure of complex petroleum substances at both local and regional scales has been predicted using the PETRORISK model (Redman, et al. 2010c) based on the principles of the hydrocarbon block method and using fate factors derived from EUSES v2.
Hydrolysis:
Hydrolysis is a reaction in which a water molecule or hydroxide ion substitutes for another atom or group of atoms present in a chemical resulting in a structural change of that chemical. Potentially hydrolysable groups include alkyl halides, amides, carbamates, carboxylic acid esters and lactones, epoxides, phosphate esters, and sulphonic acid esters (Neely and Blau, 1985). The lack of a suitable leaving group renders compounds resistant to hydrolysis.
The chemical constituents that comprise the heavy fuel oil (HFO) category consist entirely of carbon and hydrogen and do not contain hydrolysable groups. As such, they have a very low potential to hydrolyze. Therefore, this degradative process will not contribute to their removal from the environment.
The available data and available weight of evidence demonstrate that heavy fuel oils are resistant to hydrolysis because they lack a functional group that is hydrolytically reactive. Therefore, this fate process will not contribute to a measurable degradative loss of these substances from the environment. Further testing is not required under Annex XI, section 1.2.
Biodegradation:
Biodegradation in water, screening test:
Substance is a hydrocarbon UVCB. Test results for biodegradation in water are used for classification. For the purpose of risk assessment, this endpoint is characterized using quantitative structure property relationships for representative hydrocarbon structures that comprise the hydrocarbon blocks. The environmental risk of this substance is assessed using the PETRORISK model (see Product Library in PETRORISK spreadsheet attached to IUCLID Section 13).
Biodegradation in water and sediment, simulation test:
Substance is a hydrocarbon UVCB. Standard tests for this endpoint are intended for single substances and are not appropriate for this complex substance. However, this endpoint is characterized using quantitative structure property relationships for representative hydrocarbon structures that comprise the hydrocarbon blocks used to assess the environmental risk of this substance with the PETRORISK model (see Product Library tab in PETRORISK spreadsheet attached in IUCLID section 13).
Biodegradation in soil:
Substance is a hydrocarbon UVCB. Standard tests for this endpoint are intended for single substances and are not appropriate for this complex substance. However, this endpoint is characterized using quantitative structure property relationships for representative hydrocarbon structures that comprise the hydrocarbon blocks used to assess the environmental risk of this substance with the PETRORISK model (see Product Library in PETRORISK spreadsheet attached to IUCLID Section 13).
Bioaccumulation:
Aquatic / sediment bioaccumulation:
Substance is a hydrocarbon UVCB. Standard tests for this endpoint are intended for single substances and are not appropriate for this complex substance. However, this endpoint has been calculated for representative hydrocarbon structures using the BCFWIN v2.16 model within EPISuite 3.12 as input to the hydrocarbon block method incorporated into the PETRORISK model. The predicted BCFs for hydrocarbons are generally overly conservative since biotransformation is not quantitatively taken into account. Therefore, indirect exposure and resulting risk estimates predicted by PETRORISK are likely to be overestimated. For the purposes of PBT assessment, measured bioaccumulation data for representative hydrocarbon constituents have been used as detailed in Section 13, attachment "Evaluation of PBT for Petroleum Hydrocarbons".
Terrestrial bioaccumulation:
Substance is a hydrocarbon UVCB. Standard tests for this endpoint are intended for single substances and are not appropriate for this complex substance. However, this endpoint has been calculated for representative hydrocarbon structures using default algorithms in the EUSES model as input to the hydrocarbon block method incorporated into the PETRORISK model. The predicted BCFs for hydrocarbons are generally overly conservative since biotransformation is not quantitatively taken into account. Therefore, indirect exposure and resulting risk estimates predicted by PETRORISK are likely to be overestimated.
Adsorption/desorption:
Substance is a hydrocarbon UVCB. Standard tests for this endpoint are intended for single substances and are not appropriate for this complex substance. However, this endpoint is characterized for representative hydrocarbon structures that comprise the hydrocarbon blocks used to assess the environmental risk of this substance with the PETRORISK model (see library tab in PETRORISK spreadsheet attached in IUCLID section 13).
Information on Registered Substances comes from registration dossiers which have been assigned a registration number. The assignment of a registration number does however not guarantee that the information in the dossier is correct or that the dossier is compliant with Regulation (EC) No 1907/2006 (the REACH Regulation). This information has not been reviewed or verified by the Agency or any other authority. The content is subject to change without prior notice.
Reproduction or further distribution of this information may be subject to copyright protection. Use of the information without obtaining the permission from the owner(s) of the respective information might violate the rights of the owner.
