Registration Dossier
Registration Dossier
Data platform availability banner - registered substances factsheets
Please be aware that this old REACH registration data factsheet is no longer maintained; it remains frozen as of 19th May 2023.
The new ECHA CHEM database has been released by ECHA, and it now contains all REACH registration data. There are more details on the transition of ECHA's published data to ECHA CHEM here.
Diss Factsheets
Use of this information is subject to copyright laws and may require the permission of the owner of the information, as described in the ECHA Legal Notice.
EC number: 203-080-7 | CAS number: 103-11-7
- Life Cycle description
- Uses advised against
- Endpoint summary
- Appearance / physical state / colour
- Melting point / freezing point
- Boiling point
- Density
- Particle size distribution (Granulometry)
- Vapour pressure
- Partition coefficient
- Water solubility
- Solubility in organic solvents / fat solubility
- Surface tension
- Flash point
- Auto flammability
- Flammability
- Explosiveness
- Oxidising properties
- Oxidation reduction potential
- Stability in organic solvents and identity of relevant degradation products
- Storage stability and reactivity towards container material
- Stability: thermal, sunlight, metals
- pH
- Dissociation constant
- Viscosity
- Additional physico-chemical information
- Additional physico-chemical properties of nanomaterials
- Nanomaterial agglomeration / aggregation
- Nanomaterial crystalline phase
- Nanomaterial crystallite and grain size
- Nanomaterial aspect ratio / shape
- Nanomaterial specific surface area
- Nanomaterial Zeta potential
- Nanomaterial surface chemistry
- Nanomaterial dustiness
- Nanomaterial porosity
- Nanomaterial pour density
- Nanomaterial photocatalytic activity
- Nanomaterial radical formation potential
- Nanomaterial catalytic activity
- Endpoint summary
- Stability
- Biodegradation
- Bioaccumulation
- Transport and distribution
- Environmental data
- Additional information on environmental fate and behaviour
- Ecotoxicological Summary
- Aquatic toxicity
- Endpoint summary
- Short-term toxicity to fish
- Long-term toxicity to fish
- Short-term toxicity to aquatic invertebrates
- Long-term toxicity to aquatic invertebrates
- Toxicity to aquatic algae and cyanobacteria
- Toxicity to aquatic plants other than algae
- Toxicity to microorganisms
- Endocrine disrupter testing in aquatic vertebrates – in vivo
- Toxicity to other aquatic organisms
- Sediment toxicity
- Terrestrial toxicity
- Biological effects monitoring
- Biotransformation and kinetics
- Additional ecotoxological information
- Toxicological Summary
- Toxicokinetics, metabolism and distribution
- Acute Toxicity
- Irritation / corrosion
- Sensitisation
- Repeated dose toxicity
- Genetic toxicity
- Carcinogenicity
- Toxicity to reproduction
- Specific investigations
- Exposure related observations in humans
- Toxic effects on livestock and pets
- Additional toxicological data
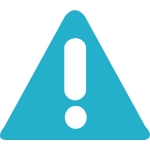
Endpoint summary
Administrative data
Link to relevant study record(s)
- Endpoint:
- basic toxicokinetics in vivo
- Type of information:
- experimental study
- Adequacy of study:
- key study
- Reliability:
- 1 (reliable without restriction)
- Rationale for reliability incl. deficiencies:
- test procedure in accordance with generally accepted scientific standards and described in sufficient detail
- Reason / purpose for cross-reference:
- reference to same study
- Objective of study:
- metabolism
- Principles of method if other than guideline:
- Two groups of rats were administered either radioactive 14C-2-ethylhexyl acrylate or 14C-2-ethylhexanol, via oral gavage, at equal molar dose levels. Repetitive blood samples were taken at the Cmax, ½ Cmax, and 1/5Cmax. Comparative metabolite profiling was performed on these collected representative blood samples.
- GLP compliance:
- yes
- Remarks:
- The study conduct, data, protocol, protocol deviation(s)/amendment(s) if applicable, and final report were inspected by the Quality Assurance Unit
- Radiolabelling:
- yes
- Species:
- rat
- Strain:
- Fischer 344/DuCrj
- Sex:
- male
- Details on test animals or test system and environmental conditions:
- TEST ANIMALS
- All rats were obtained already cannulated in the jugular vein (JVC)
- Source: Charles River (Kingston, New York)
- Age at study initiation: 9 weeks
- Weight at study initiation: 134-168 g
- Housing: single housed in glass Roth-type metabolism cages
- Diet (e.g. ad libitum): LabDiet Certified Rodent Diet #5002 (PMI Nutrition International, St. Louis, Missouri)
- Water (e.g. ad libitum): municipal water
- Acclimation period: at least five days
ENVIRONMENTAL CONDITIONS
- Temperature (°C): 20-26
- Humidity (%): 36-87
- Air changes (per hr): no data
- Photoperiod (hrs dark / hrs light): 12/12 - Route of administration:
- oral: gavage
- Vehicle:
- propylene glycol
- Details on exposure:
- PREPARATION OF DOSING SOLUTIONS:
- Dose Preparation
The equal molar levels of both radiolabeled- and unlabeled-test material were added to propylene glycol (PG) for dose preparation. The dose preparation was administered at a target volume of ~5 mL/kg bw; the target radioactivity was ~500 µCi/kg bw.
- Dose Confirmation and Homogeneity of Dose Solutions
Dose confirmation was determined by gas chromatography with a flame ionization detector (GC/FID). LSS analysis of aliquots of the 14C-labeled dose preparation taken from various locations in the vial (top, middle and bottom) was used to confirm the concentration of radioactivity and the homogeneity of the 14C-2-ethylhexyl acrylate and 14C-2-ethylhexanol. The respective dose preparations for Groups 1 to 4, described in study design, had their radiochemical homogeneity determined prior to administration (via LSS). Concentrations of test material-derived radioactivity in blood and excreta were based on analytically determined test material concentrations in dose formulations.
The measured concentration of total test material in each dose solution was 98.5% of the target concentration. The concentration of radioactivity in each of the dose solutions was within 85-100% of the target radioactivity. - Duration and frequency of treatment / exposure:
- single administration
- Dose / conc.:
- 100 mg/kg bw/day (actual dose received)
- Remarks:
- 14C-2-ethylhexyl acrylate and 2-ethylhexyl acrylate (0.054 mol/kg bw) (group 3)
- Dose / conc.:
- 70.6 mg/kg bw/day (actual dose received)
- Remarks:
- 14C-2-ethylhexanol and 2-ethylhexanol.(0.054 mol/kg bw) (group 3)
- No. of animals per sex per dose / concentration:
- 1
- Control animals:
- yes, concurrent vehicle
- Details on study design:
- - Dose selection rationale:
A dose level of 100 mg/kg body weight (bw) for 2-ethylhexyl acrylate and 70.6 mg/kg bw for 2-ethylhexanol was used in this study. These two dose levels were equimolar (0.054 mol/kg bw) based on the study design. The dose selection was based on the previous metabolism study of 2-ethylhexyl acrylate (Sapota, 1988). - Details on dosing and sampling:
- TOXICOKINETIC / PHARMACOKINETIC STUDY (Absorption, distribution, excretion)
See the linked RSS of the same study.
METABOLITE CHARACTERISATION STUDIES
- Tissues and body fluids sampled (delete / add / specify): blood
- Time and frequency of sampling: Cmax (0.17 hr), 1/2Cmax (1 hr) and 1/5Cmax (12 hr),
- From how many animals: 1
- Method type(s) for identification: Extracts of the selected blood samples were analyzed (profiled) via HPLC separation with radioactivity monitoring (RAM) detection or fraction collection (20 second fractions of eluent collected post-column) followed by liquid scintillation spectrometry (LSS) assay of the fractions
- Limits of detection: 50 dpm
TREATMENT FOR CLEAVAGE OF CONJUGATES (if applicable): no - Metabolites identified:
- yes
- Details on metabolites:
- Similar metabolite profiles from Cmax (0.17 hr), 1/2Cmax (1 hr) and 1/5Cmax (12 hr) blood samples from rats administered 14C-2-EHA or 14C-2-EH were observed. No detectable 14C-2-EHA levels were found in any Cmax (0.17 hr), 1/2Cmax (1 hr) or 1/5Cmax (12 hr) blood samples. 14C-2-EH was the only major metabolite observed in all Cmax or 1/2Cmax blood samples.
- Conclusions:
- Similar metabolite profiles from Cmax (0.17 hr), 1/2Cmax (1 hr) and 1/5Cmax (12 hr) blood samples from rats administered 14C-2-EHA or 14C-2-EH were observed. No detectable 14C-2-EHA levels were found in any Cmax (0.17 hr), 1/2Cmax (1 hr) or 1/5Cmax (12 hr) blood samples. 14C-2-EH was the only major metabolite observed in all Cmax or 1/2Cmax blood samples.
- Executive summary:
2-ethylhexyl acrylate (2-EHA) is expected to undergo rapid ester hydrolysis to form 2-ethylhexanol (2-EH) in vivo. To support this hypothesis of 2-EHA to 2-EH, the metabolism of 14C-2-ethylhexyl acrylate (2-EHA) or 14C-2-ethylhexanol (2-EH) were compared following a single oral dose administration via gavage. Male F344/DuCrl rats (one/test compound) were dosed with 2-EHA and 2-EH at molar equivalents (100 or 70.6 mg/kg body weight of 2-EHA and 2-EH, respectively), and blood samples were collected at Cmax (0.17 hr), 1/2Cmax (1 hr) and 1/5Cmax (12 hr) and representative blood samples were profiled. Similar metabolite profiles were observed. No detectable 14C-2-EHA levels were found in any blood samples. 14C-2-EH was the only major metabolite observed in all Cmax or 1/2Cmax blood samples. These study results support a common metabolic pathway with 2-EH after oral gavage administration of 2-EHA or 2-EH in rats.
- Endpoint:
- basic toxicokinetics in vivo
- Type of information:
- experimental study
- Adequacy of study:
- key study
- Reliability:
- 1 (reliable without restriction)
- Rationale for reliability incl. deficiencies:
- test procedure in accordance with generally accepted scientific standards and described in sufficient detail
- Reason / purpose for cross-reference:
- reference to same study
- Objective of study:
- excretion
- metabolism
- toxicokinetics
- Principles of method if other than guideline:
- Two groups of rats were administered either radioactive 14C-2-ethylhexyl acrylate or 14C-2-ethylhexanol, via oral gavage, at equal molar dose levels and equal molar levels of radioactivities. Repetitive blood samples were taken to determine blood 14C concentration-time course and to calculate TK parameters (absorption and elimination half-lives of the total radioactivity in blood, as well as area-under-the-curve (AUC)). During this stage, urine and feces were collected and urinary and fecal excretion of both test materials was also calculated based on the radioactivity of the samples. Expired volatiles and CO2 were also monitored.
- GLP compliance:
- yes
- Remarks:
- The study conduct, data, protocol, protocol deviation(s)/amendment(s) if applicable, and final report were inspected by the Quality Assurance Unit
- Radiolabelling:
- yes
- Species:
- rat
- Strain:
- Fischer 344/DuCrj
- Sex:
- male
- Details on test animals or test system and environmental conditions:
- TEST ANIMALS
- All rats were obtained already cannulated in the jugular vein (JVC)
- Source: Charles River (Kingston, New York)
- Age at study initiation: 9 weeks
- Weight at study initiation: 134-168 g
- Housing: single housed in glass Roth-type metabolism cages
- Diet (e.g. ad libitum): LabDiet Certified Rodent Diet #5002 (PMI Nutrition International, St. Louis, Missouri)
- Water (e.g. ad libitum): municipal water
- Acclimation period: at least five days
ENVIRONMENTAL CONDITIONS
- Temperature (°C): 20-26
- Humidity (%): 36-87
- Air changes (per hr): no data
- Photoperiod (hrs dark / hrs light): 12/12 - Route of administration:
- oral: gavage
- Vehicle:
- propylene glycol
- Details on exposure:
- PREPARATION OF DOSING SOLUTIONS:
- Dose Preparation
The equal molar levels of both radiolabeled- and unlabeled-test material were added to propylene glycol (PG) for dose preparation. The dose preparation was administered at a target volume of ~5 mL/kg bw; the target radioactivity was ~500 µCi/kg bw.
- Dose Confirmation and Homogeneity of Dose Solutions
Dose confirmation was determined by gas chromatography with a flame ionization detector (GC/FID). LSS analysis of aliquots of the 14C-labeled dose preparation taken from various locations in the vial (top, middle and bottom) was used to confirm the concentration of radioactivity and the homogeneity of the 14C-2-ethylhexyl acrylate and 14C-2-ethylhexanol. The respective dose preparations for Groups 1 to 4, described in study design, had their radiochemical homogeneity determined prior to administration (via LSS). Concentrations of test material-derived radioactivity in blood and excreta were based on analytically determined test material concentrations in dose formulations.
The measured concentration of total test material in each dose solution was 98.5% of the target concentration. The concentration of radioactivity in each of the dose solutions was within 85-100% of the target radioactivity. - Duration and frequency of treatment / exposure:
- single administration
- Dose / conc.:
- 100 mg/kg bw/day (actual dose received)
- Remarks:
- 14C-2-ethylhexyl acrylate and 2-ethylhexyl acrylate (0.054 mol/kg bw) (group 1)
- Dose / conc.:
- 70.6 mg/kg bw/day (actual dose received)
- Remarks:
- 14C-2-ethylhexanol and 2-ethylhexanol.(0.054 mol/kg bw) (group 2)
- No. of animals per sex per dose / concentration:
- 3 for time-course blood
- Control animals:
- yes, concurrent vehicle
- Details on study design:
- - Dose selection rationale:
A dose level of 100 mg/kg body weight (bw) for 2-ethylhexyl acrylate and 70.6 mg/kg bw for 2-ethylhexanol was used in this study. These two dose levels were equimolar (0.054 mol/kg bw) based on the study design. The dose selection was based on the previous metabolism study of 2-ethylhexyl acrylate (Sapota, 1988). - Details on dosing and sampling:
- TOXICOKINETIC / PHARMACOKINETIC STUDY (Absorption, distribution, excretion)
- Tissues and body fluids sampled: urine, faeces, blood, air, cage washes,
- Time and frequency of sampling:
Blood: 0.08, 0.17, 0.25, 0.5, 1, 2, 3, 6, 12, and 24h as well as every 24 hours for 7 days post-dosing (approximately 0.1-0.2 mL blood). The blood was oxidized and analyzed for radioactivity via LSS.
Urine: 12- and 24-hour post-dosing followed by 24-hour intervals for the remainder of the study. Urine samples were analyzed for radioactivity via LSS.
Feces: 24-hour intervals up to termination (168 hours post-dosing). Feces samples were analyzed for radioactivity via LSS.
Expired Volatiles: Air was drawn through the cage at approximately 850 ml/minute. The air exiting the cage was passed through charcoal to trap expired volatiles. These charcoal traps were changed at 24-hour intervals. Weighed aliquots of the charcoal were oxidized and analyzed for radioactivity via LSS.
Expired CO2: following the charcoal traps (described above) the expired air was passed through a solution of monoethanolamine:1-methoxy-2-propanol (3:7 v/v) to trap expired CO2 and analyzed for radioactivity via LSS. The CO2 traps were changed at the 6-, 12- and 24-hour time points followed by 24-hour intervals for the remainder of the study. A weighed aliquot of the final cage wash was analyzed for radioactivity via LSS.
Final Cage Wash: following the terminal sacrifice of the animals, a final cage wash (FCW) was performed with water and detergent followed by a final rinse with solvent (acetonitrile). Aweighed aliquot of the final cage wash was analyzed for radioactivity via LSS.
- Terminal Blood Samples and Sacrifice
Termination was 7 days post-dosing. Blood was obtained at sacrifice via cardiac puncture. An aliquot of the blood was directly oxidized and analyzed for radioactivity via LSS. - Statistics:
- Descriptive statistics were used, i.e., mean ± standard deviation, when applicable. All calculations in the database were conducted using Microsoft Excel (Microsoft Corporation, Redmond, Washington) spreadsheets and databases in full precision mode (15 digits of accuracy). Certain pharmacokinetic parameters were calculated for blood data, including AUC (area-under-the-curve), Cmax, 1/2Cmax and 1/5Cmax and elimination rate constants, using a pharmacokinetic computer modeling program PK Solutions (v.2.0.6., Summit Research Services, Montrose, Colorado).
- Type:
- excretion
- Results:
- Urine (0-168h): 59.37 +/- 5.2 % 2EHA; 65.71 +/- 4.4% 2EH
- Type:
- excretion
- Results:
- Feces (0-168h): 20.94 +/- 6.18% 2EHA; 17.28 +/- 2.85 % 2EH
- Type:
- excretion
- Results:
- Air (CO2) (0-168h): 12.59 +/- 0.33%; 10.59 +/- 1.02% 2EH
- Type:
- excretion
- Results:
- Total recovery (0-168h): 93.86 +/- 1.6% 2EHA; 96.13 +/- 1.23% 2EH
- Toxicokinetic parameters:
- Tmax: 0.28 +/- 0.21 h
- Remarks:
- (1st pic) 2-EHA
- Toxicokinetic parameters:
- Cmax: 18.67 +/- 24.04 µg/g
- Remarks:
- (1st pic) 2EHA
- Toxicokinetic parameters:
- Tmax: 6.00 h (2nd pic) 2EHA
- Toxicokinetic parameters:
- Cmax: 6.42 +/- 0.57 µg/g
- Remarks:
- (2nd pic) 2EHA
- Toxicokinetic parameters:
- half-life 1st: 6.23 +/- 0.65 h
- Remarks:
- 2-EHA
- Toxicokinetic parameters:
- AUC: 249.47 +/- 10.79 µg h g-1)
- Remarks:
- 2EHA
- Toxicokinetic parameters:
- Tmax: 0.25 +/- 0.22 h
- Remarks:
- (1st pic) 2EH
- Toxicokinetic parameters:
- Cmax: 27.14 +/- 8.92 µg/g
- Remarks:
- (1st pic) 2EH
- Toxicokinetic parameters:
- Tmax: 6.00 h
- Remarks:
- (2nd pic) 2EH
- Toxicokinetic parameters:
- Cmax: 4.47 +/- 0.32 µg/g
- Remarks:
- (2nd pic) 2EH
- Toxicokinetic parameters:
- half-life 1st: 5.53 +/- 0.39
- Remarks:
- 2EH
- Toxicokinetic parameters:
- AUC: 178.43 +/- 16.54
- Remarks:
- 2EH (adjusted according to 100% targeted radioactivity)
- Metabolites identified:
- no
- Conclusions:
- Blood time-courses from both 2-EHA and 2-EH were similar, with enterohepatic recirculation being observed for both substances. Half-life time (t1/2) for absorption and elimination were also similar, but Tmax and Cmax showed variability amongst animals, especially for 2-EHA. Similar mass balance (urine, CO2 and feces) and metabolite profiles from Cmax (0.17 hr), 1/2Cmax (1 hr) and 1/5Cmax (12 hr) blood samples from rats administered 14C-2-EHA or 14C-2-EH were observed in this current study.
- Executive summary:
The compound 2-ethylhexyl acrylate (2-EHA) is expected to undergo rapid ester hydrolysis to form 2-ethylhexanol (2-EH) in vivo. To support this hypothesis of 2-EHA to 2-EH, the absorption and excretion of 14C-2-ethylhexyl acrylate (2-EHA) or 14C-2-ethylhexanol (2-EH) were compared following a single oral dose administration via gavage. Both compounds were labeled at the same 14C-labeled position on the 2-ethylhexyl group. Two groups of 3 male F344/DuCrl rats were dosed at molar equivalents (100 or 70.6 mg/kg body weight of 2-EHA and 2-EH, respectively). The major pharmacokinetic parameters resulting from the blood time-courses and excretion (in urine, feces, volatiles and CO2) were determined.
Blood time courses from both 2-EHA and 2-EH were similar, with enterohepatic recirculation being observed for both substances. Half-life time (t1/2) for absorption and elimination were also similar, but Tmax and Cmax showed variability amongst animals, especially for 2-EHA. Similar mass balance (urine, CO2 and feces) were observed. These study results support a common metabolic pathway with 2-EH after oral gavage administration of 2-EHA or 2-EH in rats.
- Endpoint:
- basic toxicokinetics in vitro / ex vivo
- Type of information:
- experimental study
- Adequacy of study:
- key study
- Reliability:
- 1 (reliable without restriction)
- Rationale for reliability incl. deficiencies:
- test procedure in accordance with generally accepted scientific standards and described in sufficient detail
- Objective of study:
- metabolism
- other: Hydrolysis and glutathione conjugaison
- Principles of method if other than guideline:
- ln Vitro Hydrolysis in rat liver microsomes and whole rat blood and glutathione conjugation rates in the presence of glutathione transferases (GST).
- GLP compliance:
- no
- Specific details on test material used for the study:
- Other test substances:
- Glacial Acrylic Acid (AA)
Chemical Name: 2-Propenoic acid
Synonyms Acrylic acid
Lot/Reference/Batch Number: A829I25023
Purity: 99.69 wt%
- Methyl Acrylate (MA)
Chemical Name: 2-Propenoic acid methyl ester
Lot/Reference/Batch Number: SHBH6895
Purity: 99.7 wt%
- Ethyl Acrylate (EA)
Chemical Name: 2-Propenoic acid ethyl ester
Lot/Reference/Batch Number: A829H7K000
Purity: 99.9 wt%
- n-Butyl acrylate (BA)
Chemical Name: 2-Propenoic acid butyl ester
Synonyms: Butyl acrylate, BA - 15 PPM MEHQ (4-Methoxyphenol)
Lot/Reference/Batch Number: A829H7D000
Purity: 99.70 wt%
- iso-Butyl Acrylate (iso-BA)
Chemical Name: 2-Propenoic acid, 2-methylpropyl ester
Lot/Reference/Batch Number: 08520136W0
Purity: 99.67 wt%
- tert-Butyl Acrylate (tert-BA)
Chemical Name: 2-Propenoic acid 1,1-dimethylethyl ester
Lot/Reference/Batch Number: SHBJ2365
Purity: 99.9 wt%
- Hydroxyethyl Acrylate (HEA)
Chemical Name: 2-Propenoic acid 2-hydroxyethyl ester
Lot/Reference/Batch Number: A022HC4006
Purity: 97.52 wt%
- Hydroxypropyl Acrylate (HPA)
Chemical Name: 2-Propenoic acid monoester with 1,2-propanediol
Lot/Reference/Batch Number: A022H6F007
Purity: 97.87 wt%
- 2-Propylheptyl Acrylate (2-PHA)
Chemical Name: 2-Propenoic acid 2-propylheptyl ester
Lot/Reference/Batch Number: 0017077376
Purity/Characterization: The non-GLP certificate of analysis lists the water content as 0.02% - Radiolabelling:
- yes
- Remarks:
- Deuterium labeled acrylates were obtained for each acrylate and were used as internal standards.
- Species:
- rat
- Strain:
- Fischer 344
- Route of administration:
- other: in vitro
- Positive control reference chemical:
- Hydroxyethyl Acrylate (HEA) was used as the positive control for microsomal and blood incubations to verify the enzyme hydrolysis activity in both microsomes and blood. This material was utilized during each run of incubations.
- Details on study design:
- This study was conducted in two stages. The first stage (Stage I) comprised of test material incubation in two different matrices, either liver microsomes or whole blood from F344 rats. Whole blood was obtained from Bioreclamation, LLC (Hicksville, New York, USA) and liver microsomes were obtained from Sekisui Xenotech, LCC (Kansas City, Kansas, USA). The second phase of the study (Stage II) explored glutathione activity via incubation of the test material with tritiated glutathione/glutathione in the presence of glutathione transferase with subsequent chemical analysis.
Stage I – Acrylate Hydrolysis in Liver Microsomes and Blood from F344 Rats
Stage IA – Incubation with Rat Liver Microsomes or Whole Blood
The rat liver microsomes (20 mg/mL) were removed from -80°C storage and thawed on wet ice. Blood was removed from cold storage (4°C) and also placed on wet ice. Microsomal incubations and blood incubations were conducted according to Text Table 1. In consideration of the extreme volatility of some of these acrylates, one incubation per time point was conducted in separate 4-mL (1 dram) glass vials with Teflon-seal caps to avoid exposing a volatile sample to air at multiple time points. For liver microsomal incubations, an aliquot (10 µL) of each diluted acrylate dose solution (50 mM) was added to incubation buffer (0.1 M phosphate, pH 7.4) containing 1 mg/mL of rat liver microsomes and NADPH (1.3 mM) for a final concentration of 500 µM of acrylate with 1% or less of organic solvent. For blood incubations, an aliquot (10 µL) of the diluted acrylate dose solution (50 mM) was added to 990 µL of rat blood for a final concentration of 500 µM of acrylate with 1% or less of organic solvent. For both sets of incubations, after the addition of the test material the whole system with the Teflon-seal cap was incubated at 37°C in a vortex incubator. After incubation, each incubation sample was removed from the incubator and put into an ice-bath, and 50 µL of trifluoroacetic acid (TFA) was added to the incubation. After quickly vortexing, 1 mL (for microsomal incubation) or 2 mL (for blood incubation) of ethyl acetate containing the corresponding deuterated acrylate (i.e., internal standard; IS), was added to the incubation sample. After centrifugation for 10 minutes at 2000 rpm, the resulting supernatant (top organic layer) was transferred to a clean GC glass vial and quantitatively analyzed for remaining parent compound and the hydrolysis metabolite (AA) by gas chromatography and tandem mass spectrometry (GC/MS-MS) according to the method described below.
Stage IB – Determination of Enzymatic Kinetic Constants (Km and Vmax) for Ester Hydrolysis of Acrylates in Rat Liver Microsomes
Based on the Phase IA results, five concentrations for each acrylate were selected (31.25, 62.5, 125, 250, 500 µM) and incubated with rat liver microsomes
(0.5 mg/mL or 0.1 mg/mL) and NADPH (1.3 mM) for 10 minutes in 0.1 M phospahate buffer (pH 7.4).
The rat liver microsomes were removed from -80°C and thawed on wet ice. The microsomes were diluted 2-fold or 10-fold with cold 0.1 M phosphate buffer (pH 7.4) to afford a microsome concentration of 10 mg/mL or 2 mg/mL. The diluted microsome solution was kept cold on wet ice. The incubations were conducted according to Text Table 2 and the conditions described in Stage IA. The test material was dissolved in ACN and introduced into the rat liver microsomes in a volume of 1% of the total incubation volume (10 µL). For each acrylate, 2 replicates per concentration were incubated in the presence of microsomes and cofactor (NADPH) under physiological conditions (37°C). After incubation, each sample was removed from the incubator and put into an ice-bath, and 50 µL of TFA was added to the incubation. After quickly vortexing, 1 mL of the appropriate IS (internal standard) was added to the incubation samples. After centrifugation for 10 minutes at 2000 rpm, the resulting supernatant (top organic layer) was transferred to a clean GC glass vial and quantitatively analyzed for the hydrolysis metabolite AA by GC/MS-MS according to the method described below.
Stage II – Glutathione Reactivity
All acrylates were incubated individually with appropriate amounts of tritiated glutathione (10 µCi, 47.8 Ci/mmol) and non-tritiated glutathione (1000 µM) with the same substrate molar concentration (100 µM) in the presence of glutathione transferase (GST) (1 mg/mL) or absence of GST under physiological conditions in 0.1 M phosphate buffer (pH 7.4). After an incubation period of 60 minutes, 50 µL of TFA was added to the incubation buffer to stabilize any potential GSH conjugates. The whole solution was transferred to a 2-mL microcentrifuge tube and centrifuged for 10 minutes at 15000 rcf. The resulting supernatant was transferred to a clean glass autosampler vial for analysis. Qualitatively these samples were analyzed for glutathione conjugates via high performance liquid chromatography with time-of-flight mass spectrometry (HPLC/Q- TOF/MS-MS) and quantitatively via HPLC with eluent fractions collected. Based on the radioactive peaks observed in the reconstructed radiochromatograms from the corresponding incubations, the relative rate of GSH-conjugate formation was measured. - Details on dosing and sampling:
- Test Material Dose Stock Solutions
Dose Solution
A stock solution (500 mM) of each test material was prepared on each day of use and diluted 10-fold in acetonitrile (ACN) to a concentration of 50 mM based on the water solubility of each individual test material. Final incubations were administered as a substrate concentration equivalent to 500 µM of the applicable acrylate and had a total organic solvent content of 1% or less.
Solubility
Based on the experimental water solubility, the maximum substrate concentration (500 µM) was used for incubations for all acrylates.
Stability
The stability of the test materials was not performed as test materials were prepared within 24 hours of use.
Positive Control Dose Stock Solution
Dose Solution
Due to non-volatility and similar reactivity, HEA was used as the positive control. A stock solution (500 mM in acetonitrile) of the positive control was prepared on each day of use with a 10-fold dilution in ACN to form 50 mM based on water solubility. Final incubations were administered as a substrate concentration equivalent to 500 µM and had a total organic solvent content of 1% or less.
Stability
Stability was not performed for dose solutions as the dose solutions were used within 24 hours of preparation.
Sample Analysis
Method A: GC/MS and GC/MS-MS Analysis of Acrylates and Acrylic acid
All GC/MS and GC–MS/MS analyses were performed using an Agilent 7890A gas chromatograph (Agilent Technologies, Santa Clara, CA, USA) coupled to a triple quadrupole mass spectrometer Agilent 7000B MS (Agilent Technologies) operated in electron ionization (EI) mode.
The GC system was equipped with an Agilent 7693A autosampler (Agilent Technologies). For the separation, a Phenonemex 7HM-G009-22; ZB-FFAP column (15 m_0.18 mm i.d._0.07 mm film thickness; Phenomenex) was used. The triple quadrupole was operated either in Q1 full scan mode or in multiple reaction monitoring (MRM) mode for detecting the transitions for all analytes (acrylates and AA). The GC/MS and GC/MS-MS methods were used during the study and the optimized conditions of GC set-up and MRM transitions for each analyte and for each GC/MS-MS method are summarized in Text Table 3.
Method B: HPLC/RAM (Fraction Collection) Analysis of Acrylate GSH conjugates
The HPLC system with eluent fractions comprised of an Agilent HP 1200 HPLC system (Agilent, Palo Alto, California) and LCJet Pro (AIM Research Company, Hockessin, Delaware, USA) fraction collector. The HPLC system was equipped with a pump, an autosampler, a column compartment, a degasser, and an UV detector. The analytes were separated on Phenomenex Luna Omega 1.6 µm, Polar C18, 2.1 x 100 mm column (Phenomenex, California, USA). The components were eluted with a gradient mode at a flow rate of 0.4 mL/minute. Mobile phases consisted of ultrapure water with 0.1% formic acid (A) and acetonitrile with 0.1% formic acid (B). The gradient elution run lasted 20 minutes (0-5 minutes 0.00% B; 5-16 minutes 90% B; 16-18 minutes, 90% B; 18-18.2 minutes, 0.00% B;
18.2-20 minutes, 0.00% B). The LC eluent was introduced into a 96-well plate fraction collector (LCJet Pro) at 10 second intervals. After collection, the well plates were dried at room temperature and counted for radioactivity for each fraction with a PerkinElmer MicroBeta2 (6-dectector) top-count system (Waltham, Massachusetts, USA). The individual fraction radioactivities of each fraction were combined to reconstruct an HPLC/RAM chromatogram.
Method C: HPLC-QTOF/MS-MS Analysis of Acrylate GSH conjugates
A HPLC-QTOF/MS-MS system comprised of a Sciex API 5500 QTOF mass spectrometer (Concord, Ontario, Canada) coupled to an Agilent HP 1200 HPLC system (Agilent, Palo Alto, California) was used. The HPLC system was equipped with a pump, an autosampler, a column compartment, a degasser, and an UV detector. The analytes were separated on Phenomenex Luna@ Omega 1.6 µm, Polar C18, 2.1 x 100 mm column (Phenomenex, CA, USA). The components were eluted with a gradient mode at a flow rate of 0.4 mL/minute. Mobile phases consisted of ultrapure water with 0.1% formic acid (A) and acetonitrile with 0.1% formic acid (B). The gradient elution run lasted 20 minutes (0-5 minutes 0.00% B; 5-16 minutes 90% B; 16-18 minutes, 90% B; 18-18.2 minutes, 0.00% B; 18.2-
20 minutes, 0.00% B). The eluent from the HPLC was diverted to waste for the first 2 minutes of the run using a Valco valve from Valco Instrument Co. (Houston, Texas). The LC eluent was introduced into an electrospray ion source. Mass spectrometry was performed on the Triple TOF5600 (AB SCIEX, Foster City, CA, USA) a hybrid triple quadrupole time–of–flight mass spectrometer equipped with an electrospray (ESI) source. The system was operated with Analyst TF 1.6 software (AB SCIEX). The conditions of the MS/MS detector were as follows: first ion source gas 40 psi, second ion source gas 40 psi, curtain gas 25 psi, temperature 500ºC, ion spray voltage floating 4500 V, collision energy 35 V, collision energy spread 15 V, declustering potential 80 V. The TOF–MS range was set at m/z 50- 1500 and product ions mass range was set at m/z 50–1250. Both positive and negative ion modes were used for compound ionization. Nitrogen was used as nebulizer and auxiliary gas.
Statistics and Calculations
Descriptive statistics were used, i.e., mean ± standard deviation, or relative standard deviation (standard deviation/mean). All calculations were conducted using Microsoft Excel spreadsheets in full precision mode (15 digits of accuracy). The half-life values of the acrylates and the rates of hydrolysis to AA for the nine acrylates in whole rat blood and rat liver microsomes in Stage IA were determined using GraphPad Prism version
5.03 (GraphPad Software, La Jolla, CA, USA). The percent remaining of the parent esters were fit to one-phase exponential decay curves to determine ke (min-1) and Clint (µL/min/mg). Calculation of Km and Vmax for the AA formation from acrylate in rat liver microsmes (Stage IB) was also performed using GraphPad Prism v5.03 according to the Michaelis-Menten Kinetic Model - Type:
- other: Rat Liver Microsomal Incubations
- Results:
- Ke from parent (min): 0.306; Half-Life from parent (min): 2.26; Ke from AA formation (min): 0.244; Half-Life from AA formation (min): 2.83
- Type:
- other: Rat Blood Incubations
- Results:
- Ke from parent (min): 0.180; Half-Life from parent (min): 3.85; Ke from AA formation (min): 0.179; Half-Life from AA formation (min): 3.87
- Type:
- other: GSH incubations in the presence of GST
- Results:
- Rate formation (nmol/mg protein.min): 1.73
- Conclusions:
- Nine acrylates, including methyl acrylate (MA), ethyl acrylate (EA), butyl acrylate (BA), iso-butyl acrylate (iso-BA), tert-butyl acrylate (tert-BA), 2-hydroxyethyl acrylate (HEA), 2- hydroxypropyl acrylate (HPA), 2-ethylhexyl acrylate (2-EHA), and 2-propylheptyl acrylate (2-PHA), were chosen for initial experimental determination of metabolism rates in rat liver microsomes and whole rat blood at a single substrate concentration (500 µM). Additionally,
Km and Vmax determinations were made by performing incubations utilizing various concentrations (32.25, 62.5, 125, 250, and 500 µM) and a single rat liver microsomal protein concentration of 0.1 mg/mL or 0.5 mg/mL. After rates were determined, a third set of incubations were performed to evaluate the ability of each acrylate to conjugate with glutathione in the presence of glutathione transferases (GST).
In rat liver microsomes, eight acrylate esters (excluding tert-BA) were hydrolyzed to form the metabolite acrylic acid (AA). For some acrylates (i.e., EA, BA, iso-BA), the half-life values calculated from the concentration of the remaining parent acrylate were comparable to the half-life values calculated from the parent acrylate concentration calculated from AA formation. The small acrylates (MA, EA, BA, iso-BA) and the large acrylate (2-EHA) have a half-life of less than 8.5 minutes (0.77-8.2 min); HEA, HPA, and 2-PHA have half-life values of ranging from 20 minutes to 61 minutes. Under the rat liver microsomal conditions, tert-BA is relatively stable.
In whole rat blood, all nine acrylate esters are rapidly metabolized, shown by a significant and (nearly) complete loss of the parent acrylate. However, unlike the rat liver microsomal incubations, the concentrations of the formed AA were significantly lower. In fact, except the background levels of AA, no significant AA was observed in all blood incubations of MA, tert-BA, HEA, and HPA. Thus, despite significant loss in parent acrylates, there is not a corresponding formation of AA in blood. Based on the measured concentrations of the remaning parent acrylates, the half-life values for all acrylates were less than 12 minutes in rat blood (0.99-11min).
In the serial substrate concentrations (31.25, 62.5, 125, 250, and 500 µM) of acrylate esters, further experiments were conducted to determine Km and Vmax values for acrylate ester hydrolysis to AA in rat liver microsomes. Km and Vmax values were determined for all acrylate esters except HEA and tert-BA.
All nine acrylates formed a single GSH conjugate in the presence of GST. The rate of formation of these GSH conjugates ranged from 1.2 to 3.94 nmol/mg protein/min.
Overall, these in vitro metabolism results imply that all nine acrylate esters can be quickly metabolized through hydrolysis to AA and/or glutathione conjugation in vivo. - Executive summary:
The hydrolysis and glutathione conjugation rates of methyl acrylate (MA), ethyl acrylate (EA), butyl acrylate (BA), iso-butyl acrylate (iso-BA), tert-butyl acrylate (tert-BA), 2-hydroxyethyl acrylate (HEA), 2-hydroxypropyl acrylate (HPA), 2-ethylhexyl acrylate (2-EHA), and 2-propylheptyl acrylate (2-PHA), were evaluated in vitro. Initial experimental determination of metabolism rates was performed with rat liver microsomes and whole rat blood at a single substrate concentration (500 µM). Additionally, Km and Vmax determinations were made by performing incubations utilizing various concentrations (32.25, 62.5, 125, 250, and 500 µM) and a single rat liver microsomal protein concentration of 0.1 mg/mL or 0.5 mg/mL. After rates were determined, a third set of incubations were performed to evaluate the ability of each acrylate to conjugate with glutathione in the presence of glutathione transferases (GST). In rat liver microsomes, eight acrylate esters (excluding tert-BA) were hydrolyzed to form the metabolite acrylic acid (AA). In whole rat blood, all nine acrylate esters are rapidly metabolized. Km and Vmax values were determined for seven of nine acrylate esters excluding HEA and tert-BA. All nine acrylates formed a single GSH conjugate in the presence of GST. The half-life values and elmination rates in both rat microsomal and blood incubation conditions and GSH conjugation rates in the presence of GST for all acrylates, are summarized in the following Table.
Incubation Types
Rat Liver Microsomal Incubations
Rat Blood Incubations
GSH incubations in the presence of GST
Compound Name
Ke from parent (min)
Half-Life from parent (min)
Ke from AA formation (min)
Half-Life from AA formation (min)
Ke from parent (min)
Half Life from parent compound (min)
Ke from AA fromation (min)
Half Life from AA formation (min)
Rate formation (nmol/mg protein.min)
MA (Methyl Acrylate)
0.0845
8.20
0.121
5.73
0.465
1.49
ND
ND
1.67
EA (Ethyl Acrylate)
0.372
1.87
0.385
1.80
0.303
2.29
ND
ND
2.19
BA (n-Butyl Acrylate)
0.771
0.770
0.711
0.710
0.297
2.33
0.259
2.68
3.94
iso-BA (i-Butyl Acrylate)
0.866
0.798
0.778
0.888
0.281
2.47
0.250
2.77
2.28
tert-BA (tert-Butyl Acrylate)
ND
ND
ND
ND
0.0941
7.37
ND
ND
1.83
HEA (2-Hydroxyethyl Acrylate)
0.0089
61.1
0.0111
62.4
0.271
2.56
ND
ND
1.87
HPA (Hydroxypropyl Acrylate)
0.0295
23.5
0.0231
30.0
0.704
0.985
ND
ND
2.35
2-EHA (2-Ethylhexyl Acrylate)
0.306
2.26
0.244
2.83
0.180
3.85
0.179
3.87
1.73
2-PHA (2-Propylheptyl Acrylate)
0.0336
20.6
0.0456
15.2
0.0617
11.2
0.0585
11.8
1.20
Ke: Elimination rate.
Overall, these in vitro metabolism results indicate that all nine acrylate esters can be quickly metabolized primarily through hydrolysis to AA and/or glutathione conjugation in vivo.
Referenceopen allclose all
Metabolite Profiling
The LC/RAM chromatograms of extracts of Cmax (0.17 hr), 1/2Cmax (1 hr) and 1/5Cmax (12 hr) blood samples collected from rats administered with 14C-2- ethylhexyl acrylate or 14C-2-ethylhexanol are shown in Figure 1 and Figure 2, respectively. As shown in Figures 1 and 2, only two peaks (Peak a and Peak b) were detected from the blood samples collected from rats administered either 14C-2-EHA or 14C-2-EH. Peak a eluted at 1.5 min and Peak b eluted at 9.5 min. The retention time of both peaks did not match the retention time of 14C-2-EHA standard (Figure 1A and Figure 2A); however, the retention time of peak b matched the retention time of the 14C- 2-EH standard, supporting that 14C-2-EHA was quickly hydrolyzed to 14C-2-EH in rats after oral gavage administration of 14C-2-EHA. It also should be noted that the slight retention time difference between peak b and 14C-2-EH standard in chromatograms (Figures 1A-1B and Figures 2A-2B) is due to the LC/RAM analysis methods, where both 14C-2-EHA or 14C-2-EH standards were analyzed via HPLC with direct radioactivity monitoring and the blood samples were analyzed via HPLC separation with fraction collection (20 second fractions of eluent collected post-column) followed by liquid scintillation spectrometry (LSS) assay of the fractions. Peak a was a second metabolite related to 14C-2-EH metabolism as it is seen in chromatograms in both Figures 1B, 1C and Figures 2B, 2C. Similar metabolite profiles were obtained from Cmax (0.17 hr) blood samples of 14C-2-EHA and 14C-2-EH [(Figure 1B and Figure 2B); No detectable metabolite peak(s) were found in 1/5Cmax (12 hr) blood samples (Figure 1D and Figure 2D). The 1/2Cmax (1 hr) blood samples (Figure 1C and Figure 2C) had peak b detected in 1/2Cmax (1 hr) blood sample of 14C-2-EH, but not detected in 1/2Cmax (12 hr) blood sample of 14C-2-EHA. Peak b did not appear with either 14C-2-EH or 14C-2-EHA at 1/5Cmax (Figure 1D and Figure 2D). These metabolite profiling results further support the assumption that 14C-2-EHA was hydrolyzed to 14C-2-EH in vivo, resulting in similar pharmacokinetics between 14C-2-EHA and 14C-2-EH as discussed above.
In-life Parameters
There were no signs of toxicity observed in any animals following oral administration of 14C-2-ethylhexyl acrylate or 14C-2-ethylhexanol.
Time-Course Concentration of Radioactivity and Pharmacokinetic Parameters in Blood
Blood concentrations of 2-EHA or 2-EH equivalents from Group 1 and 2 were detectable over the entire study collection interval of 0.08 to 168 hours post-dosing (Table 3A).
The blood time courses from both 2-EHA and EH treated rats (Group 1 and Group 2) demonstrated EHC (enterohepatic recirculation) (Table 3A), indicating that 2-EHA and 2-EH have similar pharmacokinetics in rats after a single oral gavage dose. As shown in Table 3A, the individual blood concentration at earlier time points (0.08 through 2 hours) are variable, especially for animals administered 2-EHA. This difference could be caused by animal variability, especially for the 2-EHA dose group (Table 3A). The Tmax and Cmax differences from Group 1 and Group 2 are due to the animal variability. Based on further pharmacokinetic analyses of the blood time courses, the major pharmacokinetic parameters for both 2-EHA and 2-EH were calculated using PK Solutions (a pharmacokinetic computer modeling program, version 2.0.6., Summit Research Services, Montrose, Colorado) and are summarized in Table 4. As shown in Table 4, pharmacokinetic parameters from individual animals are very similar except first peak Tmax, Cmax and AUC. This difference could be caused by animal variability, especially for the 2-EHA dose group (Table 3A). As the 14C-labeled position in 2-EHA was in the 2-ethylhexyl group (the same position as in 14C-labeled 2-EH), the similarity of PK parameters from both 2-EHA and 2-EH indicated that 2-EHA was quickly hydrolyzed to 2-EH in rats after oral gavage dosage and resulted in similar pharmacokinetics to 2-EH.
Excretion Radioactive Doses of 2-EHA or 2-EH
After a single oral dose of 14C-2-EHA (at 100 mg/kg) or 14C-2-EH (at 70.6 mg/kg) in male rats, the recovery values of radioactive dose in urine, feces, blood, charcoal and CO2 trapping solution collected at different time points, are shown in Table 5. As shown in Table 5, the total average recoveries from both 2-EHA and 2-EH are similar (94% for 2-EHA and 96% for 2-EH). The corresponding recoveries from urine, feces and CO2 trapping solution at each collection time point are very similar for both 2-EHA and 2-EH (Table 5). Especially, the majority of radioactivity of CO2 which was recovered in the first 24 hours post-dosing are similar for both 2-EHA and 2-EH, supporting this hypothesis that 2-EHA was hydrolyzed in the rat to form 2-EH, which was further metabolized to radioactive CO2.
Stage I – Acrylate Hydrolysis in Rat Liver Microsomes and Blood from F344 Rats
Stage IA–Incubation with Rat Liver Microsomes or Rat Blood (Tables 1, 2; figure 1)
As shown Table 1 and Figure 1, the similarity in the graph lines (measured vs calculated) indicate that all acrylates except tert-BA were metabolized by rat liver microsomes in the presence or absence of NADPH to form the hydrolysis metabolite, AA. Without microsomes, all acrylates were relatively stable under the incubation conditions, indicating the hydrolysis of acrylates was mainly catalyzed by the enzymes contained in rat liver microsomes. At the same time points of 0, 30, or 60 min, the concentrations of the remaining acrylates and the acrylates calculated from the formation of AA are very comparable between the microsomal incubations with NADPH and the microsmal incubations without NADPH, implying that the hydrolysis of acrylate in rat liver microsomes is mainly due to the esterases which do not require NADPH for the enzymatic hydrolysis of acrylates.
In some cases, as shown in Table 4, the half-life values calculated from the concentration of the remaining parent acrylate are comparable to the half-life values calculated from the concentration of parent acrylate based on AA formed in the microsomal incubations (i.e., EA, BA, iso-BA). According to the half-life values, acrylates (MA, EA, BA, iso-BA, and 2-EHA) have a half-life of less than
8.5 minutes (0.77-8.2 min). Under the same incubation conditions, HEA, HPA, and 2-PHA have half-lives of longer than 15 minutes, but less than 62 minutes. Possibly due to the steric hindrance, tert-BA is relatively stable under the same microsomal incubation conditions.
TABLE 4. Elimination Rates, Intrinsic Clearance and Half-life in Rat Liver Microsomes and Whole Rat Blood for Acrylates at 500µM Substrate Concentration
|
Rat Liver Microsomal Incubation |
Rat Blood Incubation |
||||||||||
Compound Name |
Clint from parent (µl/min/mg) |
Ke from parent (min) |
Half-Life from parent (min) |
Clint from AA formation (µl/min/mg) |
Ke from AA formation (min) |
Half-Life from AA formation (min) |
Clint from parent (µl/min) |
Ke from parent (min) |
Half Life from parent compound (min) |
Clint from AA formation (µl/min/mg) |
Ke from AA fromation (min) |
Half Life from AA formation (min) |
MA (Methyl Acrylate) |
84.5 |
0.0845 |
8.20 |
121 |
0.121 |
5.73 |
465 |
0.465 |
1.49 |
ND |
ND |
ND |
EA (Ethyl Acrylate) |
372 |
0.372 |
1.87 |
385 |
0.385 |
1.80 |
303 |
0.303 |
2.29 |
ND |
ND |
ND |
BA (n-Butyl Acrylate) |
771 |
0.771 |
0.770 |
711 |
0.711 |
0.710 |
297 |
0.297 |
2.33 |
259 |
0.259 |
2.68 |
iso-BA (i-Butyl Acrylate) |
866 |
0.866 |
0.798 |
778 |
0.778 |
0.888 |
281 |
0.281 |
2.47 |
250 |
0.250 |
2.77 |
tert-BA (tert-Butyl Acrylate) |
ND |
ND |
ND |
ND |
ND |
ND |
94.1 |
0.0941 |
7.37 |
ND |
ND |
ND |
HEA (2-Hydroxyethyl Acrylate) |
11.3 |
0.0113 |
61.1 |
8.14 |
0.00814 |
85.2 |
271 |
0.271 |
2.56 |
ND |
ND |
ND |
HPA (Hydroxypropyl Acrylate) |
29.5 |
0.0295 |
23.5 |
23.1 |
0.0231 |
30.0 |
704 |
0.704 |
0.985 |
ND |
ND |
ND |
2-EHA (2-Ethylhexyl Acrylate) |
306 |
0.306 |
2.26 |
244 |
0.244 |
2.83 |
180 |
0.180 |
3.85 |
179 |
0.179 |
3.87 |
2-PHA (2-Propylheptyl Acrylate) |
33.6 |
0.0336 |
20.6 |
45.6 |
0.0456 |
15.2 |
61.7 |
0.0617 |
11.2 |
58.5 |
0.0585 |
11.8 |
ND: not determined due to no AA formation or much less AA formation Ke: elimination rate; Clint in microsomal incubation: Intrinsic clearance (Ke x volume of incubation/mg/mL microsomal protein) Clint in blood incubation: Intrinsic clearance (Ke x volume of incubation) |
Stage IA–Incubation with Pooled Rat Blood (Table 3, Figure2)
As shown Table 3 and Figure 2, all acrylates are metabolized in rat blood. However, unlike rat liver microsomal incubations, where the concentrations of the acrylate calculated from the formed AA correlated well with the remaining corresponding acrylates, the acrylate concentration calculated from AA formation in rat blood incubations are significantly different from the concentrations of the remaining corresponding acrylates (Figure 2). In fact, AA was not quantifiable from the blood incubations of MA, tert-BA, and HPA except the background level of AA (Table 3). To investigate whether the non-quantifiable AA in some of the blood incubations of acrylates was due to the stability of AA in rat blood, a rat blood time-course of AA was conducted and the result indicated that AA was relatively stable under rat blood incubation conditions (Figure 2,AA blood time course). As shown in Figure 2, AA is relatively stable in rat blood over a 60-minute period. This stability study result clearly showed that the AA was either not formed or formed at considerably lower levels than the corresponding rat liver microsomal incubations for the acrylates (MA, EA, BA, iso-BA, tert-BA, HEA, and HPA) during the rat blood incubations.
As shown in Table 4, the half-life values for all acrylates are less than 12 minutes (0.99-11.2 min), implying that all acrylate esters are quickly metabolized in rat blood. Whether measured by loss of parent or calculated from AA formation when possible (ie. BA, iso-BA, 2-EHA and 2-PHA), half-life values were similar in rat blood.
Stage IB–Determination of Enzymatic Kinetics (Kmand Vmax) of Ester Hydrolysis of Acrylates in Rat Liver Microsomes (Table 5, figure 3)
The final concentrations of the formed metabolite AA in the corresponding microsomal incubations at the microsomal protein level of 0.5 mg/ml, are summarized in Table 5. Based on the concentrations of AA and the substrate concentrations, the initial Michaelis-Menten kinetic plots were depicted in Figure 3. As shown in Figure 3, due the stability of tert-BA in the rat liver microsomal incubation conditions, tert- BA was hardly hydrolyzed and therfore, Km and Vmax values could not be calculated.
At the same microsomal protein level of 0.5 mg/mL, MA, HPA, HEA, and 2-PHA were partially hydrolyzed and their corresponding Km and Vmax values were able to be calculated and summarized in Table 7 except HEA which did not show Michaelis-Menten kinetics.
EA, BA, iso-BA, and 2-EHA were almost 100% hydrolyzed at each substrate concentration (Table 5), indicating that microsmal enzymes were not saturated by the substrate concentration at the current levels, therfore, no Kmand Vmaxvalues could be calculated for EA, BA, iso-BA, and 2-EHA at the microsomal protein concentration level of 0.5 mg/mL. In order to saturate the microsomal enzymes at the same range of substrate concentrations, the microsomal protein level was reduced to 0.1 mg/mL for EA, BA, iso-BA, and 2-EHA. At this new microsomal protein level, all incubations and analyses were repeated for EA, BA, iso-BA, 2- EHA, and MA. While Kmand Vmaxvalues were determined for MA at the higher microsomal protein concentration, this compound was included in the second analysis to verify similar performance across the assays. The final results and Michaelis-Menten kinetic plots were shown in Table 6 and Figure 4, respectively. As shown in Figure 4, at this lower level of microsomal protein, EA, BA, iso-BA, 2-EHA, and MA exhibited Michaelis-Menten kinetics; therefore their corresponding Kmand Vmaxvalues were able to be calculated and summarized in Table 7. The Kmand Vmaxvalues for MA were comparable (see study files); therefore, averaged values are shown in Table 7.
TABLE 7. Hydrolysis Rates of Acrylates in Rat Liver Microsomes
Test Material |
Vmax (µM)a |
Vmax (nmol/min/mg) |
Km (µM)a |
MAb |
512 |
216 |
2002 |
EA |
410 |
410 |
1157 |
BA |
788 |
788 |
731 |
iso-BA |
1188 |
1188 |
1293 |
HEA |
ND |
ND |
ND |
HPAc |
847 |
169 |
829 |
2-EHA |
602 |
602 |
503 |
2-PHAc |
538 |
108 |
979 |
a: V max and Km were calculated by GraphPad Prism based on the Michaelis-Menten kinetic model b: Averaged values from both microsomal protein concentrations (0.1 and 0.5 mg/mL) c: V max and Km were obtained from rat liver microsmal incubation at 0.5 mg/mL ND: Km and Vmax cannot be determined based on Michaelis-Menten kinetic model |
Stage II–Glutathione Reactivity
According to the experimental procedure described above, each acrylate was incubated with GSH and tritiated GSH. After centrifugation, the resulting supernatants were analyzed by the HPLC with fractions collection (Method B) and HPLC/Q-TOF/MS analysis (Method C) for acrylate GSH (Acrylate-SG) conjugate formation (detailed analytical procedures are included in the study files). The final reconstructed radiochromatograms for all acrylate esters are depicted in Figure 5. As shown in Figure 5, all acrylate esters can react with GSH in the presence of GST to form one major peak (one adduct) in addition to the GSH peak. Under the same conditions, AA does not form GSH adduct. Further HPLC/Q-TOF/MS analysis of the new peak showed it was an Acrylate-SG adduct (see study files) (Table 8). Using the peak percentage of each GSH conjugate, the ratio of cold GSH to tritiated GSH, and the specific activity of tritiated GSH, the total GSH conjugate and rate formation of the GSH conjugate are summarized in Table 8. Overall, the rates of formation of the GSH conjugates in the presence of GST for all the acrylates ranged from 1.20 to 3.94 nmol/mg protein/min. Without GST, the rate of GSH conjugation of 2-EHA was slower than the GSH conjugation rate in the presence of GST.
TABLE 8. Formation of Acrylate GSH conjugates from incubation of acrylate with GSH in the presence of GST
Substrate name |
Substrate concentration (µM) |
Cold GSH concentration (µM) |
Cold GSH concentration (µmmol) |
Tritiated GSH (hot GSH)(µCi)a |
Formed GSH Conjugate |
Retention time in HPLC/RAM |
Detected m/z of [M+H]+ in high resolution MS |
Calculated m/z of [M+H]+ based on the conjugate structure |
Peak Area (percetage) |
Total triated GSH conjugate (µmol) |
Total cold GSH conjugate (µmol)b |
Total GSH conjugate (µmol) |
Rate formation (nmol/mg protein.min) |
AA |
100 |
1000 |
1 |
10 |
AA-SG |
ND |
ND |
378.1342 |
NDc |
ND |
ND |
ND |
ND |
MA |
MA-SG |
7.50 |
394.1277 |
394.1278 |
8.31 |
0.0174 |
0.0831 |
0.100 |
1.67 |
||||
EA |
EA-SG |
8.20 |
408.1430 |
408.1435 |
10.9 |
0.0228 |
0.109 |
0.132 |
2.19 |
||||
BA |
BA-SG |
9.30 |
436.1738 |
436.1748 |
19.6 |
0.0409 |
0.196 |
0.237 |
3.94 |
||||
iso-BA |
Iso-BA-SG |
9.30 |
436.1746 |
436.1748 |
11.3 |
0.0236 |
0.113 |
0.137 |
2.28 |
||||
tert-BA |
tert-BA-SG |
9.20 |
436.174 |
436.1748 |
9.06 |
0.0190 |
0.091 |
0.110 |
1.83 |
||||
HEA |
HEA-SG |
8.10 |
424.1376 |
424.1284 |
9.29 |
0.0194 |
0.0929 |
0.112 |
1.87 |
||||
HPA |
HPA-SG |
8.30 |
438.1525 |
438.154 |
11.7 |
0.0244 |
0.116 |
0.141 |
2.35 |
||||
2-EHA |
2-EHA-SG |
11.2 |
492.2362 |
492.2374 |
8.59 |
0.0180 |
0.0859 |
0.104 |
1.73 |
||||
2-EHA (no GST) |
2-EHA-SG |
11.2 |
492.2362 |
492.2374 |
5.15 |
0.0108 |
0.0515 |
0.0623 |
1.04 |
||||
2-PHA |
2-PHA-SG |
12.0 |
520.2692 |
520.2687 |
5.96 |
0.0125 |
0.0596 |
0.0721 |
1.20 |
||||
a:Specific activity of hot GSH: 48.7 Ci/mmol; b: based on the ratio (4.78) of cold GSH versus hot GSH ; c: Not detected in HPLC/RAM, but detedted in HPLC/Q-TOF/MS; ND: Not detected based on the Low limit quantitation (LLQ) of 50 CPM |
Overall, the half-life values and elmination rates in both rat microsomal and blood incubation conditions and GSH conjugation rates in the presence of GST for all acrylates, are summarized in Table 9.
TABLE 9. Summary of Half-life Values, Elimination Rates, and Rates of GSH Conjugation
Incubation Types |
Rat Liver Microsomal Incubations |
Rat Blood Incubations |
GSH incubations in the presence of GST |
||||||
Compound Name |
Ke from parent (min) |
Half-Life from parent (min) |
Ke from AA formation (min) |
Half-Life from AA formation (min) |
Ke from parent (min) |
Half Life from parent compound (min) |
Ke from AA fromation (min) |
Half Life from AA formation (min) |
Rate formation (nmol/mg protein.min) |
MA (Methyl Acrylate) |
0.0845 |
8.20 |
0.121 |
5.73 |
0.465 |
1.49 |
ND |
ND |
1.67 |
EA (Ethyl Acrylate) |
0.372 |
1.87 |
0.385 |
1.80 |
0.303 |
2.29 |
ND |
ND |
2.19 |
BA (n-Butyl Acrylate) |
0.771 |
0.770 |
0.711 |
0.710 |
0.297 |
2.33 |
0.259 |
2.68 |
3.94 |
iso-BA (i-Butyl Acrylate) |
0.866 |
0.798 |
0.778 |
0.888 |
0.281 |
2.47 |
0.250 |
2.77 |
2.28 |
tert-BA (tert-Butyl Acrylate) |
ND |
ND |
ND |
ND |
0.0941 |
7.37 |
ND |
ND |
1.83 |
HEA (2-Hydroxyethyl Acrylate) |
0.0089 |
61.1 |
0.0111 |
62.4 |
0.271 |
2.56 |
ND |
ND |
1.87 |
HPA (Hydroxypropyl Acrylate) |
0.0295 |
23.5 |
0.0231 |
30.0 |
0.704 |
0.985 |
ND |
ND |
2.35 |
2-EHA (2-Ethylhexyl Acrylate) |
0.306 |
2.26 |
0.244 |
2.83 |
0.180 |
3.85 |
0.179 |
3.87 |
1.73 |
2-PHA (2-Propylheptyl Acrylate) |
0.0336 |
20.6 |
0.0456 |
15.2 |
0.0617 |
11.2 |
0.0585 |
11.8 |
1.20 |
Ke: Elimination rate. |
Description of key information
Key value for chemical safety assessment
- Bioaccumulation potential:
- no bioaccumulation potential
- Absorption rate - oral (%):
- 100
- Absorption rate - dermal (%):
- 10
- Absorption rate - inhalation (%):
- 100
Additional information
Absorption, excretion.
Inhalation exposure
Urinary excretion of metabolites following inhalational exposure indicates absorption of 2-ethylhexyl acrylate occurring by this route (Vodicka et al., 1986, 1990).
A slight dose related increase in the urinary excretion of thioethers was observed in rats exposed to air concentrations of 2-EHA ranging from 250 mg/m3 to 1000 mg/m3 (33 to 133 ppm) (Vodicka et al., 1986).
Male Wistar rats exposed by 6 hours inhalation to 2-EHA in concentrations from 250 to 4800 mg/m3 over 24 hours excreted thioethers in urine in a dose dependent manner decreasing from 8.0 to 3.0% (at 1000 mg/m3) of the dose of 2-EHA indicating saturable metabolism along this pathway. Dose related depletion of non-protein SH groups in blood, liver and brain was seen at concentrations of and above 2400 mg/m3 (Vodicka et al. 1990).
2-EHA is a substance with a low volatility, its vapor pressure is 24 Pa at 25°C. It is a lipophilic compound (log P >4) with a low water solubility (9.6 mg/L) and it may be taken up by micellular solubilisation.
Therefore, according to the REACH Guidance, a default inhalation absorption rate of 100% will be used for risk assessment.
Oral exposure
Excretion of a radioactive marked dose following oral exposure indicate absorption of 2-EHA occurring by this route (Sapota, 1988; Zhang et al., 2017).
14C-2-EHA (labelled on the vinyl carbons) was administered p.o. on rats (100 mg/kg bw) (Sapota, 1988). Within the first 72 hours 93% of the dose had been excreted, 50% in expired air, 41% in urine, and 1% in faeces.
The absorption and excretion of 14C--2-EHA) and 14C-2-ethylhexanol (14C-2-EH) were evaluated following a single oral dose administration via gavage (Zhang et al., 2017). Two groups of 3 male F344/DuCrl rats were dosed at molar equivalents (100 or 70.6 mg/kg body weight of 2-EHA and 2-EH, respectively). The excretions in urine, feces and air (volatiles and CO2) were determined. The total average recoveries (0-168 h) was 94% and 96 %, respectively. The recoveries (0-24 h) from urine, feces and CO2 trapping solution were 56, 18 and 11% for 14C-2-EHA and 63, 16 and 9% for 14C-2-EH, respectively.
While high dose absorption studies with 2-ethylhexyl acrylate have not been performed, 2-ethylhexanol at high doses has been demonstrated to undergo saturation of metabolism following oral bolus dosing. Excretion balance studies were conducted with 2-ethylhexanol (2-EH) in female Fischer 344 rats following single high (500 mg/kg) and low (50 mg/kg) oral doses of [14C]-2-EH, following repeated oral dosing with unlabeled 2-EH at the low level, and following a 1 mg/kg i.v. dose of [14C]-2-EH (Deisinger et al., 1994). The high, low and repeated low oral dose studies with 2-EH showed similar excretion balance profiles of [14C], with some evidence of metabolic saturation at the high dose. No evidence of metabolic induction was seen following the repeated low oral dosing. All of the oral doses were eliminated rapidly, predominantly in the urine during the first 24 h following dosing. Urinary metabolites eliminated were predominately glucuronides of oxidized metabolites of 2-EH, including glucuronides of 2-ethyladipic acid, 2-ethylhexanoic acid, 5-hydroxy-2-ethylhexanoic acid and 6-hydroxy-2-ethylhexanoic acid.
Therefore, according to the REACH Guidance, a default oral absorption rate of 100% will be used for risk assessment.
Dermal exposure
No experimental data are available.
The substance must be sufficiently soluble in water to partition from the stratum corneum into the epidermis. Between 1-100 mg/l absorption is anticipated to be low to moderate. With a log P above 4, the rate of penetration may be limited by the rate of transfer between the stratum corneum and the epidermis, but uptake into the stratum corneum will be high. Therefore, the rate of absorption is estimated to be low.
The rate of absorption of 2-EHA was estimated using the IH SkinPerm model (v2.04). IH SkinPerm is a mathematical tool for estimating dermal absorption. The rate of mass build-up (or loss) on the skin comes from the deposition rate onto the skin minus the absorption rate into the Stratum Corneum (SC) and the amount evaporating from the skin to the air. An internal validation study (Régnier, 2018) have shown that compared to in vitro data, IH SkinPerm allowed the estimation of the dermal absorption rate with a good confidence and a low frequency of underestimation (2% of the liquid substances). For an instantaneous deposition of 1000 mg over 1000 cm² of skin or a deposition rate over time of 1 mg/cm²/h, the absorption rates were calculated to be 1.8% and 0.2% of the dose after 8 hours of contact, respectively.
This estimation is supported by permeation studies of methyl-, ethyl- and butyl acrylate performed with skin from new-born piglets using the same experimental set up with static diffusion cells (Schenk et al., 2018).
Therefore, according to the REACH Guidance, a default skin absorption rate of 10% will be used risk assessment.
Intraperitoneal or intravenous routes
Male Wistar rats were administrated an intravenous dose of 10 mg/kg bw or 50 mg/kg bw of (14C)-2-EHA (Cikrt et al. 1986). Biliary excretion of radioactivity was followed in 1-3 hour intervals within the first 24 hours after administration. A significant increase in bile flow (243%) was observed. In the 24-hours 2.2% of the dose was eliminated via bile at both doses, most of it (83%) during the first 3 hours.
Male Wistar rats were administrated an intraperitoneal or intravenous dose of 10 mg/kg bw of (14C)-2-EHA labelled on the vinyl carbons (Gut et al. 1988). Within the first 24 hours about 50% of the dose had been excreted in expired air, 7-13% in urine and less than 0.01% in faeces.
Male Wistar albino rats were administrated an intraperitoneal of 100 mg/kg bw of [2,3-14C]-2-EHA (Sapota 1988). Within the first 72 hours 90% of the dose had been excreted, 78% in expired air, 10% in urine, and 3% in faeces.
Distribution
Intraperitoneal route
In one study male Wistar rats were administrated an intraperitoneal dose of 10 mg/kg bw of (14C)-2-EHA labelled on the vinyl carbons (Gut et al. 1988). In another study male Wistar albino rats were administrated an intraperitoneal dose of 100 mg/kg bw of [2,3-14C]-2-EHA acrylate (Sapota 1988). In both studies plasma radioactivity concentration reached a peak level at about 2-3 hours after administration indicating easy absorption through this route. In tissues the highest concentrations of radioactivity was found in kidney, liver, spleen and the lungs. In the study with a dose of 100 mg/kg bw 6.5% of the dose was found in tissues at 3 hours after administration. The radioactivity in the tissues decreased slowly with time. At 72 hours after administration 1% of the dose was still found in the examined tissues. The radioactivity in adipose tissue and sciatic nerve was still relatively high.
Intravenous route
In one study male Wistar rats were administrated an intravenous dose of 10 mg/kg bw of (14C)-2-EHA labelled on the vinyl carbons (Gut et al. 1988). In another study male Wistar rats were administrated an intravenous dose of 10 mg/kg bw or 50 mg/kg bw of (14C)-2-EHA (Cikrt et al. 1986). The highest concentrations of radioactivity in tissues was found in kidney, liver, brain, thymus and spleen.
Metabolism
The major route of metabolism of acrylate esters, including 2-ethylhexyl acrylate involved the rapid cleavage of the ester bond by carboxylic esterases, resulting in internal exposure to AA and the corresponding alcohol. A subsequent metabolic pathway involves metabolism of AA to carbon dioxide (CO2) via the propionate degradation pathway. The respective alcohols are metabolized via either a catalase peroxidation pathway or the alcohol dehydrogenase pathway. Acrylate esters are also expected to undergo conjugation with GSH to form thioesters (Frederick et al., 1992), with the main urinary conjugate identified as N-acetyl-S-(2-carboxyethyl)cysteine. Inhibition of the hydrolytic pathway with a carboxylase inhibitor results in increased metabolism via the GSH conjugation route. There is no evidence to suggest that the vinyl moiety undergoes epoxidation. Based on a recent in vitro investigation for the hydrolysis and glutathione conjugation rates of the acrylate esters, 2-ethylhexyl acrylate and the majority of other acrylates tested were metabolized by rat liver microsomes in the presence or absence of ß-nicotinamide adenine dinucleotide 2’-phosphate reduced tetrasodium salt hydrate (NADPH) to form AA (Zhang et al., 2018). It was reported that the hydrolysis of the acrylate esters in rat liver microsomes is mainly mediated by esterases, which do not require NADPH.
In vitro hydrolysis
The hydrolysis properties of methyl-, ethyl-, n-butyl, iso-butyl-, t-butyl- and 2-ethylhexyl acrylates were determined in liver S9 fraction and blood plasma of male Wistar rats (Roos, 2015). The decrease of the acrylates and the formation of acrylic acid was determined analytically.
In liver S9 fraction, the degradation of acrylates and the formation of acrylic acid was clearly observed. The half-lives were 1.40, 0.84, 0.74 and 1.15 min for EA, n-BA, i-BA and 2-EHA, respectively. The degradation takes place almost completely via the ester cleavage. Due to the steric hindrance by the butyl group, t-BA was not hydrolysed. Due to technical difficulties for the determination of the MA content, it was possible to determine a half-life for MA. The degradation rates were 18.612, 23.790, 0.476 and 5.165 µM/g liver equivalent*min for n-BA, i-BA, t-BA and 2EHA, respectively.
The degradation times in plasma were slower than in liver S9 fraction. The half-life were 34.62, 8.45, 8.15, 6.48 min for MA, n-BA, i-BA and 2-EHA, respectively. The degradation rates of the 6 acrylates were 31.54, 77.91, 83.87, 114.32, 21.17, and 59.86 µmol/L*min, respectively. The tertiary compounds, t-BA, showed a limited hydrolysis like with the S9 fraction.
Furthermore, hydrolysis of selected acrylate esters was also investigated in an in vitro assay (Zhang et al., 2018). A group of acrylate esters (i.e., MA, EA, n-BA, i-BA, t-BA and 2-EHA) was chosen for initial experimental determination of metabolism rates in rat liver microsomes and whole rat blood at a single substrate concentration of 500 µM. The incubation was performed in combination with the presence or absence of microsomes and NADPH. All acrylate esters except t-BA were metabolised by rat liver microsomes in the presence or absence of NADPH to form AA (Table below). Without microsomes, all acrylate esters were relatively stable under the incubation conditions, indicating the hydrolysis of acrylate esters was mainly catalysed by the enzymes contained in rat liver microsomes. The concentrations of the remaining acrylate esters, both measured concentrations and the back-calculated concentrations from the formation of AA, support the similarity between the microsomal incubations regardless of the presence of NADPH. This suggests that the hydrolysis of acrylate esters in rat liver microsomes is mainly due to the esterases which do not require NADPH for the enzymatic hydrolysis of acrylate esters. The tested acrylate esters (MA, EA, n-BA, i-BA, and 2-EHA) have a half-life of less than 8.5 minutes (0.77-8.2 min) in the rat liver microsomes, indicating that metabolism is rapid. t-BA was relatively stable under the same microsomal incubation conditions, probably due to the presence of steric hindrance due to its tertiary structure. The time-course of the remaining acrylate esters, both measured and back-calculated values, showed a rapid metabolism of the acrylate esters with almost complete consumption of the acrylate esters within the culture. However, the concentrations of the formed AA were significantly lower in the rat blood compared to the microsomal culture. The half-lives for all acrylate esters, based on the measured concentrations of the remaining parent acrylate esters, were less than 12 minutes in rat blood, ranging 0.99 – 11.2 minutes. Overall, the rate of hydrolysis of the acrylate esters increased in the order butyl > ethyl > methyl. Both studies showed slower hydrolysis rates of acrylate esters in whole rat blood than in the rat liver microsomes. The hydrolysis rate of t-BA was slower than other acrylate esters included in the assays as consistently demonstrated in both studies. A variation in the hydrolysis rate was noted for MA between the two assays.
Table. Kinetic values of acrylate esters in rat liver microsomes and in rat whole blood (Zhang, 2018)
Substance |
Rat Liver Microsomal Incubation |
Rat Blood Incubation |
Hydrolysis Rate#1 |
||||
Half-Life (min) |
Half-Life (min) |
Vmax#2 |
Vmax |
Km |
|||
Parent |
Based on AA formation |
Parent |
Based on AA formation |
||||
MA |
8.20 |
5.73 |
1.49 |
- |
512#3 |
216#3 |
2002#3 |
EA |
1.87 |
1.80 |
2.29 |
- |
410 |
410 |
1157 |
nBA |
0.77 |
0.71 |
2.33 |
2.68 |
788 |
788 |
731 |
iBA |
0.8 |
0.888 |
2.47 |
2.77 |
1188 |
1188 |
1293 |
tBA |
- |
- |
7.37 |
- |
- |
- |
- |
2EHA |
2.26 |
2.83 |
3.85 |
3.87 |
602 |
602 |
503 |
#1In rat liver microsomes.
#2Vmax and Km were calculated by GraphPad Prism based on the Michaelis-Menten kinetic model.
#3Averaged values from both microsomal protein concentrations (0.1 and 0.5 mg/mL).
In vivo metabolism
2-EHA is believed to undergo carboxylesterase-catalysed hydrolysis to 2-ethylhexanol and acrylic acid, like other acrylate esters (Cikrt et al. 1986).
To support this hypothesis of 2-EHA to 2-EH, the metabolism of 14C-2-EHA or 14C-2-ethylhexanol (2-EH) were compared following a single oral dose administration via gavage (Zhang et al., 2017). Male F344/DuCrl rats (one/test compound) were dosed with 2-EHA and 2-EH at molar equivalents (100 or 70.6 mg/kg body weight of 2-EHA and 2-EH, respectively), and blood samples were collected at Cmax (0.17 hr), 1/2Cmax (1 hr) and 1/5Cmax (12 hr) and representative blood samples were profiled. Similar metabolite profiles were observed. No detectable 14C-2-EHA levels were found in any blood samples. 14C-2-EH was the only major metabolite observed in all Cmax or 1/2Cmax blood samples. These study results support a common metabolic pathway with 2-EH after oral gavage administration of 2-EHA or 2-EH in rats.
Excretion balance studies were conducted with 2-ethylhexanol (2-EH) in female Fischer 344 rats following single high (500 mg/kg) and low (50 mg/kg) oral doses of [14C]-2-EH, following repeated oral dosing with unlabeled 2-EH at the low level, and following a 1 mg/kg i.v. dose of [14C]-2-EH (Deisinger et al., 1994). Urinary metabolites eliminated were predominately glucuronides of oxidized metabolites of 2-EH, including glucuronides of 2-ethyladipic acid, 2-ethylhexanoic acid, 5-hydroxy-2-ethylhexanoic acid and 6-hydroxy-2-ethylhexanoic acid.
The acrylic acid (AA) is decarboxylated and degraded to carbon dioxide (Black et al., 1995). C3H mice and Fischer 344 rats, respectively, were treated by gavage (40 or 150 mg/kg bw) with [1-14C]-AA. Mice rapidly absorbed and metabolised orally administered AA, with about 80% of the dose exhaled as 14CO2 within 24 h. Excretion in urine and faeces accounted for approximately 3% and 1% of the dose, respectively. Elimination of the 14C radiolabel from plasma, liver and kidney was rapid but it was slower from fat. The disposition of orally administered AA in rats was similar to the results obtained from mice. High-performance liquid chromatography (HPLC) analysis of rat urine and rat and mouse tissues indicated that absorbed AA was rapidly metabolised by the ß-oxidation pathway of propionate catabolism. No unchanged AA was detected 1 h after oral administration; however, several metabolites that were more polar than AA were measured, including 3-hydroxypropionate. Neither AA nor its metabolites were detected at later times after oral administration.
Conjugation to Glutathione
The C=C double bond of acrylate esters makes these chemicals potential Michael acceptors capable of electrophilic attack of protein and other cellular macromolecules. This is the mode of action through which a wide range of toxicities including allergic contact dermatitis is thought to be mediated. This reactivity also means that acrylate esters are capable of conjugating with cellular GSH, as evidenced in the in vitro studies (Zhang, 2017a, 2017b; 2018; Udinsky and Frederick, 1994).
In an in vitro assay, a group of acrylate esters (MA, EA, BA, i-BA, t-BA and 2-EHA) was individually incubated with tritiated- and non-tritiated glutathione in the presence or absence of GST at pH 7.4 for 60 minutes (Zhang et al., 2018). All acrylate esters can react with GSH in the presence of GST to form one major peak (Acrylate-SG adduct) in addition to the GSH peak. Under the same conditions, AA did not form GSH adduct. Overall, the rates of formation of the GSH conjugates in the presence of GST for all the acrylate esters were similar (ranging from 1.20 to 3.94 nmol/mg protein/min), suggesting the involvement of GST to conjugate GSH with the acrylate esters in the category. Based on the previous in vitro results, McCarthy et al. (1994) reported that the following order of reactivity for GST depletion: MA > nBA > EA.
Incubation Types |
GSH incubations in the presence of GST |
Compound Name |
Rate formation (nmol/mg protein.min) |
MA (Methyl Acrylate) |
1.67 |
EA (Ethyl Acrylate) |
2.19 |
BA (n-Butyl Acrylate) |
3.94 |
iso-BA (i-Butyl Acrylate) |
2.28 |
tert-BA (tert-Butyl Acrylate) |
1.83 |
2-EHA (2-Ethylhexyl Acrylate) |
1.73 |
In vivo, 2-ethylhexyl acrylate to a minor extent reacts with non-protein SH groups in for instance glutathione causing depletion of the non-protein SH groups and excretion of thioethers in urine as described in the following studies:
Male Wistar rats exposed by 6 hours inhalation to 2-ethylhexyl acrylate in concentrations from 250 to 4800 mg/m3 over 24 hours excreted thioethers in urine in a dose dependent manner decreasing from 8.0 to 3.0% (at 1000 mg/m3) of the dose of 2-ethylhexyl acrylate indicating saturable metabolism along this pathway. Dose related depletion of non-protein SH groups in blood, liver and brain was seen at concentrations of and above 2400 mg/m3. (Vodicka et al. 1990).
When male Wistar rats were administrated an intraperitoneal dose of 10 mg/kg bw of (14C)-2-EHA labelled on the vinyl carbons 2% of the dose was found as thioethers in the urine (Gut et al. 1988).
The principal eliminated metabolite in expired air was carbon dioxide in two studies in male Wistar rats given an intraperitoneal or intravenous dose of (14C)-2-EHA (Gut et al. 1988, Sapota 1988).
When 2-EHA and its metabolite acrylic acid reacts with the reduced form of glutathion (GSH), mercapturic acids can be formed (Cikrt et al. 1986).
The relative potential of methyl acrylate (MA), ethyl acrylate (EA), n-butyl acrylate (n-BA), and 2-ethylhexyl acrylate (2-EHA) to alter glutathione (GSH) concentrations and redox status of GSH in the rat forestomach was compared when administered by gavage to groups of five male F344/DuCrl rats at a single dose of 0, 17.2, 20.0, 25.6, or 36.8 mg/kg body weight (equimolar dose 0.2 mmol/kg) in corn oil. Approximately 3-hours after the administration of test materials, a time point optimized from previous studies with EA, all rats were sacrificed and the forestomach was removed, weighed, flash frozen in liquid nitrogen, and placed on dry ice until analysis for GSH and GSSG levels. The control animals received corn oil only.
The average GSH concentration was 527.5 µg/g tissue in the control animals and the GSH reduction was 57.9% and 42.9% in MA and EA treated rats, respectively. A slight decrease (6.3% reduction) in GSH concentration was observed in rats treated with 2-EHA. No appreciable change was detected in GSH concentration in rats treated with n-BA (average 535.6 µg/g tissue). The average GSSG concentration was 9.4 µg/g tissue in the control animals and the GSSG reduction was 38.1, 26.0, 11.7, and 7.5% in MA, EA, 2-EHA, and n-BA treated rats, respectively. The GSH:GSSG ratio was not substantially altered. These observations indicated the following order of GSH-depleting potency in forestomach in rats: MA > EA > 2-EHA > n-BA. However, because only one time point and one dose level were investigated, this order of GSH-depleting potency is not definitive.
Table. GSH reactivity of acrylate esters (Ji and Marshall, 2017)
Substance |
Dose level |
No. of animals |
GSH |
GSSG |
||||
mg/kg |
mmol/kg |
µg/g |
% |
µg/g |
% |
GSH:GSSG |
||
Control |
0 |
0 |
5 |
527.5 ± 108.6 |
- |
9.4 ± 1.6 |
- |
56.3 ± 5.5 |
MA |
17.2 |
0.2 |
5 |
221.9 ± 12.7 |
57.9 |
5.8 ± 1.0 |
38.1 |
39.3 ± 7.7 |
EA |
20.0 |
0.2 |
5 |
295.4 ± 114.8 |
42.9 |
6.9 ± 2.1 |
26.0 |
42.5 ± 4.6 |
nBA |
25.6 |
0.2 |
5 |
535.6 ± 136.7 |
1.5 |
8.7 ± 1.8 |
7.5 |
61.6 ± 5.3 |
2EHA |
36.8 |
0.2 |
5 |
494.2 ± 9.8 |
6.3 |
8.3 ± 1.0 |
11.7 |
60.3 ± 7.2 |
Bold= interpreted to be treatment-related
The depletion of GSH in the forestomach following a single administration of EA was also reported in male F344 rats at 50 mg/kg bw resulting in 63-84% reduction (Udinsky and Frederick, 1994*).
The depletion of GSH in the forestomach following a single administration of EA, at the dose levels of 0, 20, 50 and 100 mg/kg bw, was also reported in male C57BL/6 mice (ARTF, 2017). Based on the analysis that wasconducted approximately three hours after the dosing via oral gavage, the levels of GSH and GSSG were substantially decreased in a dose-dependent manner. The GSH depletion, relative to the concurrent vehicle control, was 52.7, 63.6 and 71.7% at 20, 50 and 100 mg/kg groups, respectively. The GSSG reduction, relative to the control, was 64.8, 76.8 and 81.3% at 20, 50 and 100 mg/kg groups, respectively. As per the finding in male F344/DuCrl rats (Ji and Marshall, 2017), the GSH:GSSG ratio was not appreciably changed in the treated animals.
Toxicokinetics
2-EHA is expected to undergo rapid ester hydrolysis to form 2-ethylhexanol (2-EH) in vivo.
To
support this hypothesis of 2-EHA to 2-EH, the absorption and excretion
of 14C-2-EHA or 14C-2-ethylhexanol (2-EH) were compared following a
single oral dose administration via gavage (Zhang et al., 2017). Both
compounds were labeled at the same 14C-labeled position on the
2-ethylhexyl group. Two groups of 3 male F344/DuCrl rats were dosed at
molar equivalents (100 or 70.6 mg/kg body weight of 2-EHA and 2-EH,
respectively). Blood sample was collected from animals at 0.08, 0.17,
0.25, 0.5, 1, 2, 3, 6, 12, and 24-hour as well as every 24 hours
post-dosing and thereafter up to the study termination (seven days
post-dosing).
The area under the curve (AUC) were identified as 249.47 µg h/g (Cmax=
18.67 ug/g, tmax= 0.28 hour) for 2-EHA and 151.67 µg h/g (Cmax= 27.14
ug/g, tmax= 0.25 hour) for 2-EH. Blood concentrations of 14C-2EHA or
14C-2EH equivalents were detectable over the entire study collection
interval of 0.08 to 168 hours post-dosing. Blood time courses from both
2-EHA and 2-EH were similar, with enterohepatic recirculation being
observed for both substances. This indicates that 2-EHA and 2-EH have
similar pharmacokinetic profiles in rats after a single oral gavage
dose. Half-life time (t1/2) for absorption and elimination were also
similar (Table), but Tmax and Cmax showed variability amongst animals at
earlier time points, especially for 2-EHA. As the14C-labelled position
in 2-EHA was in the 2-ethylhexyl group (the same position as in
14C-labelled 2-EH), the similarity of pharmacokinetic parameters from
both 2-EHA and 2-EH indicated that 2-EHA was quickly hydrolysed to 2-EH
in rats after oral gavage dosage and resulted in showing a similar
pharmacokinetic profile to 2-EH.
Table: Kinetic parameters of 2EHA and 2EH equivalents in rat blood following a single oral dose of 14C-2EHA or 14C-2EH (Zhang et al., 2017)
Test Substance |
2EHA |
2EH |
Pharmacokinetic Parameters (Based on measured 2EH labelled radioactivity) |
Mean±S.D. |
Mean±S.D. |
tmax(h) estimated from 1st peak |
0.28±0.21 |
0.25±0.22 |
Cmax(µg/g) estimated from 1st peak |
18.67±24.04 |
27.14±8.92 |
tmax(h) estimated from 2nd peak#1 |
6.00±0.00 |
6.00±0.00 |
Cmax(µg/g) estimated from 2nd peak#1 |
6.42±0.57 |
4.47±0.32 |
Absorption t½(h) |
2.83±0.37 |
2.56±1.55 |
Elimination t½a (h) |
6.23±0.65 |
5.53±0.39 |
Elimination t½ß (h) |
65.08±2.81 |
64.47±3.05 |
AUC0--168(µg h g-1) |
249.47±10.79 |
151.67±14.06 |
#1Peak a
Male Wistar rats were administrated an intraperitoneal or intravenous dose of 10 mg/kg bw of (14C)-2-EHA labelled on the vinyl carbons. Elimination of radioactivity from blood was bi-exponential. The plasma half-life for the distribution phase was 60 minutes (i.p.) and 30 minutes (i.v.) for 4 months old and 130 minutes (i.p.) and 115 minutes (i.v.) for 7 months old. For the elimination phase, the half-life was 6 hours (i.p.) and 5 hours (i.v.) for the youngest rats and 14 hours (i.p. and i.v.) for the oldest. (Gut et al. 1988).
Male Wistar albino rats were administrated an intraperitoneal dose of 100 mg/kg bw of [2,3-14C]-2-EHA . Elimination of radioactivity from blood had a monophasic character. The plasma half-life was about 22 hours. The half-life for excretion was calculated to be about 1½ hour. (Sapota 1988).
Additional references:
*Udinsky J.R., Frederick C.B. (1994). Ethyl acrylate: Tim course for the absorption from the gut, distribution to tissues of parent compound, and glutathione depletion. Unpublished data, The Dow Chemical Company.
**ARTF (2017): Investigation of the potential for acute ethyl acrylate treatment to alter glutathione concentrations and redox status in the rodent forestomach. Report number: 161011. Toxicology and Environmental Research and Consulting, the Dow Chemical Company, Michigan, United States. Owner company; Basic acrylic monomer manufacturers, Inc. and Acrylate REACH Task Force. Report date: May 11, 2017.
Information on Registered Substances comes from registration dossiers which have been assigned a registration number. The assignment of a registration number does however not guarantee that the information in the dossier is correct or that the dossier is compliant with Regulation (EC) No 1907/2006 (the REACH Regulation). This information has not been reviewed or verified by the Agency or any other authority. The content is subject to change without prior notice.
Reproduction or further distribution of this information may be subject to copyright protection. Use of the information without obtaining the permission from the owner(s) of the respective information might violate the rights of the owner.
