Registration Dossier
Registration Dossier
Data platform availability banner - registered substances factsheets
Please be aware that this old REACH registration data factsheet is no longer maintained; it remains frozen as of 19th May 2023.
The new ECHA CHEM database has been released by ECHA, and it now contains all REACH registration data. There are more details on the transition of ECHA's published data to ECHA CHEM here.
Diss Factsheets
Use of this information is subject to copyright laws and may require the permission of the owner of the information, as described in the ECHA Legal Notice.
EC number: 614-557-8 | CAS number: 68515-81-1
- Life Cycle description
- Uses advised against
- Endpoint summary
- Appearance / physical state / colour
- Melting point / freezing point
- Boiling point
- Density
- Particle size distribution (Granulometry)
- Vapour pressure
- Partition coefficient
- Water solubility
- Solubility in organic solvents / fat solubility
- Surface tension
- Flash point
- Auto flammability
- Flammability
- Explosiveness
- Oxidising properties
- Oxidation reduction potential
- Stability in organic solvents and identity of relevant degradation products
- Storage stability and reactivity towards container material
- Stability: thermal, sunlight, metals
- pH
- Dissociation constant
- Viscosity
- Additional physico-chemical information
- Additional physico-chemical properties of nanomaterials
- Nanomaterial agglomeration / aggregation
- Nanomaterial crystalline phase
- Nanomaterial crystallite and grain size
- Nanomaterial aspect ratio / shape
- Nanomaterial specific surface area
- Nanomaterial Zeta potential
- Nanomaterial surface chemistry
- Nanomaterial dustiness
- Nanomaterial porosity
- Nanomaterial pour density
- Nanomaterial photocatalytic activity
- Nanomaterial radical formation potential
- Nanomaterial catalytic activity
- Endpoint summary
- Stability
- Biodegradation
- Bioaccumulation
- Transport and distribution
- Environmental data
- Additional information on environmental fate and behaviour
- Ecotoxicological Summary
- Aquatic toxicity
- Endpoint summary
- Short-term toxicity to fish
- Long-term toxicity to fish
- Short-term toxicity to aquatic invertebrates
- Long-term toxicity to aquatic invertebrates
- Toxicity to aquatic algae and cyanobacteria
- Toxicity to aquatic plants other than algae
- Toxicity to microorganisms
- Endocrine disrupter testing in aquatic vertebrates – in vivo
- Toxicity to other aquatic organisms
- Sediment toxicity
- Terrestrial toxicity
- Biological effects monitoring
- Biotransformation and kinetics
- Additional ecotoxological information
- Toxicological Summary
- Toxicokinetics, metabolism and distribution
- Acute Toxicity
- Irritation / corrosion
- Sensitisation
- Repeated dose toxicity
- Genetic toxicity
- Carcinogenicity
- Toxicity to reproduction
- Specific investigations
- Exposure related observations in humans
- Toxic effects on livestock and pets
- Additional toxicological data
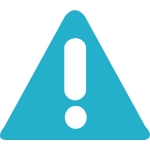
Endpoint summary
Administrative data
Link to relevant study record(s)
Description of key information
Key value for chemical safety assessment
Additional information
INTRODUCTION
1-Nonanol is acolourless, viscous liquid that has a low water solubility and a strong odour.
ABSORPTION
As an aliphatic alcohol, 1-nonanol is likely to be absorbed following exposure by all common physiological routes (dermal, oral and inhalation) (OECD, 2006). The extent of absorption of aliphatic alcohols varies with chain length. For example, in rats, the extent of dermal penetration increases up to C7 (1-heptanol) and thereafter declines with longer carbon chain lengths (Valette and Cavier, 1954). Dermal absorption studies using hairless mice, and comparative in vitro skin permeation data, show that for aliphatic alcohols with chain lengths varying from 8 to 16 carbon atoms, there is an inverse relationship between absorption and chain length with the short to intermediate chain alcohols (up to C12) having a significant absorption potential. Thus approximately 50% of a dose of radiolabelled n-[1-14C]octanol was absorbed upon skin application to mice for 24 hours, a figure that decreased with increasing chain length to about 7% for similarly labelled 1‑decanol and 2.84% for 1-dodecanol (Iwata et al. 1987).
Lipophilic substances that come into contact with the skin can readily penetrate the lipid-rich stratum corneum by passive diffusion at a rate proportional to their lipid solubility and inversely to their molecular weight (Marzulli et al. 1965).
The extent of absorption of aliphatic alcohols from the gastrointestinal tract also depends upon chain length. Although no actual data were found for 1-nonanol, short-chain aliphatic alcohols are known to be rapidly and extensively absorbed from the gastrointestinal tract (Aaes-Jorgensen et al. 1959; Bandi et al. 1971a, 1971b), whereas long-chain saturated alcohols (e.g. C18) are said to be poorly absorbed (CIR, 1985). The presence of only <0.5% of an oral dose ofthe structurally‑similar 1‑octanol in the faeces of rats (Miyazaki, 1955) supports the view that absorption of ingested 1‑nonanol is likely to be extensive.
DISTRIBUTION
Absorbed 1-nonanol potentially could be widely distributed within the body (OECD, 2006). However, its rapid and efficient metabolism and elimination from the bodymeans that 1‑nonanol is not expected to be retained or to accumulate (Bevan, 2001; OECD, 2006). Short-chain aliphatic alcohols readily penetrate the blood-brain barrier, whereas longer chain alcohols (C16-C18) cross this barrier in only trace amounts (Gelman and Gilbertson, 1975).
METABOLISM
As a primary alcohol, absorbed 1-nonanol will initially be metabolised (oxidised), primarily by alcohol dehydrogenase, to the corresponding aldehyde (nonanal). The aldehyde is a transient intermediate that is rapidly converted by further oxidation to the acid (nonanoic acid) by aldehyde dehydrogenase. Nonanoic acid is then susceptible to degradation via acyl-CoA intermediates by the mitochondrialb-oxidation process. This mechanism removes C2 units in a stepwise process. The rate ofb-oxidation tends to increase with increasing chain length (JECFA, 1999). Mice excretedmore than 90% of the absorbed dose of a similar alcohol (radiolabelled n‑[1‑14C]dodecanol) in expired air (evidently as carbon dioxide) following skin application, suggesting that metabolism of absorbed 1-nonanol would also be extensive (Iwata et al. 1987).
An alternative metabolic pathway exists through microsomal or peroxisomal degradation of the carboxylic acid metabolite (nonanoic acid) viaw- orw-1 oxidation followed by β-oxidation (Verhoeven et al. 1998). [This pathway provides an efficient route for the degradation of branched-chain alcohols.]
The acids formed from the longer-chain aliphatic alcohols can also enter lipid biosynthesis and may be incorporated in phospholipids and neutral lipids (Bandi et al. 1971a, 1971b; Mukherjee et al. 1980).
The hydroxyl function of the parent nonanol and the carboxy function of the nonanoic acid metabolite may also undergo conjugation reactions to form sulphates and/or glucuronides. For linear aliphatic alcohols, this pathway generally accounts for less than 10% of the metabolism (Kamil et al. 1953; McIsaac and Williams, 1958). In rabbits, 4.1% of an oral dose of 1-nonanol was found as urinary glucuronide (Kamil et al. 1953).
EXCRETION
Following the 24-hour application of the structurally-related 1-dodecanol (radiolabelled with14C on the 1-carbon atom) to the skin of hairless mice, more than 90% of the absorbed dose was excreted in expired air and 3.5% was eliminated in the faeces and urine by 24 hours; only 4.6% of the absorbed dose [representing 0.13% of the applied dose] remained in the body (Iwata et al. 1987). A similar general pattern of extensive and rapid excretion would be expected for 1‑nonanol.
When rats were given an oral dose of the close analogue 1-octanol, only trace amounts (<0.5%) were detected unchanged in the faeces (Miyazaki, 1955), whereas for two higher alcohols (1‑hexadecanol and 1-octadecanol) faecal recoveries of 20 and 50% were found, respectively (McIsaac and Williams, 1958; Miyazaki, 1955). The equivalent figure for 1-nonanol might be expected to be similar to that for 1‑octanol.
The glucuronic acid conjugates formed during the metabolism of most aliphatic alcohols are excreted in the urine (Wasti, 1978; Williams, 1959).For 1-nonanol, 4.1% of an oral dose was excreted by the rabbit in urine as glucuronide, compared with 9.5% for 1-octanol and 3.5% for 1‑decanol (Kamil et al. 1953).
Although lipophilic alcohols such as 1-nonanol have the physicochemical potential to accumulate in breast milk, rapid metabolism to the corresponding carboxylic acid followed by further degradation suggests that breast milk can only be, at most, a minor route of elimination from the body (OECD, 2006).
REFERENCES
Aaes-Jorgensen E, Privett OS and Holman RT (1959). Essential fatty acid activities of hydrocarbons and alcohols analogous to linoleate and linolenate. Journal of Nutrition 67, 413-421 (cited in Gelman and Gilbertson, 1975).
Bandi ZL, Mangold HK, Holmer G and Aaes-Jorgensen E (1971a). The alkyl and alk-1-enyl glycerols in the liver of rats fed long chain alcohols or alkyl glycerols. FEBS Letters 12, 217-220.
Bandi ZL, Aaes-Jorgensen E and Mangold HK (1971b). Metabolism of unusual lipids in the rat. 1. Formation of unsaturated alkyl and alk-1-enyl chains from orally administered alcohols. Biochimica et Biophysica Acta 239, 357-367.
Bevan C (2001). Monohydric Alcohols - C7 to C18, aromatic and other alcohols. Patty’s Toxicology. Eds E Bingham, B Cohrssen and CH Powell. 5th Edition, Vol. 6, J. Wiley and Sons, New York (cited in OECD, 2006).
Casarett and Doull (1991). Toxicology. The basic science of poisons. Eds MO Amdur, J Doull and CD Klassen. 4thEdition, Pergamon Press, New York.
CIR (1985). Final report on the safety assessment of stearyl alcohol, oleyl alcohol and octyl dodecanol. Journal of the American College of Toxicology 4, 1-29.
CIR (1988). Final report on the safety assessment of ceteayl alcohol, cetyl alcohol, isostearyl alcohol, myristyl alcohol and behenyl alcohol. Journal of the American College of Toxicology 7, 359-413.
FDA (1978). Monograph on stearyl alcohol. US Department of Commerce. NTIS PB-289 664. Food and Drug Administration, Washington DC.
Gelman RA and Gilbertson JA (1975). Permeability of the blood-brain barrier to long-chain alcohols from plasma. Nutrition and Metabolism 18, 169-175.
Iwata Y, Moriya Y and Kobayashi T (1987). Percutaneous absorption of aliphatic compounds. Cosmetics and Toiletries 102, 53-68.
JECFA (1999). Evaluation of certain food additives and contaminants. 49thReport of the Joint FAO/WHO Expert Committee on Food Additives. WHO Tech Rep Series No 884. WHO, Geneva.
Kamil IA, Smith JN and Williams RT (1953). Studies in detoxication 46. The metabolism of aliphatic alcohols. The glucuronic acid conjugation of acyclic aliphatic alcohols. Biochemical Journal 53, 129-136 (cited in McIsaac and Williams, 1958).
Marzulli FN, Callahan JF and Brown DW (1965). Chemical structure and skin penetrating capacity of a short series of organic phosphates and phosphoric acid. Journal of Investigative Dermatology 44, 339-344 (cited in Casarett and Doull, 1991).
McIsaac WM and Williams RT (1958). The metabolism of spermaceti. WA Journal of Biological Chemistry 2, 42-44.
MiyazakiM (1955). Nutritive value of aliphatic alcohols II. The nutritive value and toxicity of saturated alcohols of six to eighteen carbon atoms. Journal of the Agricultural and Chemical Society of Japan 29, 501-505 (cited in FDA, 1978).
Mukherjee KD, Weber N, Mangold HK et al. (1980). Competing pathways in the formation of alkyl, alk-1-enyl and acyl moieties in the lipids of mammalian tissues. European Journal of Biochemistry 107, 289-294.
OECD (2006) Long Chain Alcohols. SIDS Initial Assessment Report for SIAM 22.
Valette G and Cavier R (1954). Percutaneous absorption and chemical composition. Examples of hydrocarbons, alcohols and esters. Archives Internationale de Pharmacodynamie 97, 232-240 [in French].
Verhoeven NM, Wanders RJ, Poll-The BT, Saudubray JM and Jacobs C (1998). The metabolism of phytanic acid and pristanic acid in man. A review.Journal of Inherited and Metabolic Diseases 21, 697-728 (cited in OECD, 2006).
Wasti K (1978). A literature review – problem definition studies on selected toxic chemicals. Environmental Protection Research Division, US Army Medical Research and Development Command, Maryland USA (cited in CIR, 1988).
Williams RT (1959). Detoxification Mechanisms. 2ndEdition, Chapman and Hall,London.
Information on Registered Substances comes from registration dossiers which have been assigned a registration number. The assignment of a registration number does however not guarantee that the information in the dossier is correct or that the dossier is compliant with Regulation (EC) No 1907/2006 (the REACH Regulation). This information has not been reviewed or verified by the Agency or any other authority. The content is subject to change without prior notice.
Reproduction or further distribution of this information may be subject to copyright protection. Use of the information without obtaining the permission from the owner(s) of the respective information might violate the rights of the owner.
