Registration Dossier
Registration Dossier
Data platform availability banner - registered substances factsheets
Please be aware that this old REACH registration data factsheet is no longer maintained; it remains frozen as of 19th May 2023.
The new ECHA CHEM database has been released by ECHA, and it now contains all REACH registration data. There are more details on the transition of ECHA's published data to ECHA CHEM here.
Diss Factsheets
Use of this information is subject to copyright laws and may require the permission of the owner of the information, as described in the ECHA Legal Notice.
EC number: 238-484-2 | CAS number: 14484-64-1
- Life Cycle description
- Uses advised against
- Endpoint summary
- Appearance / physical state / colour
- Melting point / freezing point
- Boiling point
- Density
- Particle size distribution (Granulometry)
- Vapour pressure
- Partition coefficient
- Water solubility
- Solubility in organic solvents / fat solubility
- Surface tension
- Flash point
- Auto flammability
- Flammability
- Explosiveness
- Oxidising properties
- Oxidation reduction potential
- Stability in organic solvents and identity of relevant degradation products
- Storage stability and reactivity towards container material
- Stability: thermal, sunlight, metals
- pH
- Dissociation constant
- Viscosity
- Additional physico-chemical information
- Additional physico-chemical properties of nanomaterials
- Nanomaterial agglomeration / aggregation
- Nanomaterial crystalline phase
- Nanomaterial crystallite and grain size
- Nanomaterial aspect ratio / shape
- Nanomaterial specific surface area
- Nanomaterial Zeta potential
- Nanomaterial surface chemistry
- Nanomaterial dustiness
- Nanomaterial porosity
- Nanomaterial pour density
- Nanomaterial photocatalytic activity
- Nanomaterial radical formation potential
- Nanomaterial catalytic activity
- Endpoint summary
- Stability
- Biodegradation
- Bioaccumulation
- Transport and distribution
- Environmental data
- Additional information on environmental fate and behaviour
- Ecotoxicological Summary
- Aquatic toxicity
- Endpoint summary
- Short-term toxicity to fish
- Long-term toxicity to fish
- Short-term toxicity to aquatic invertebrates
- Long-term toxicity to aquatic invertebrates
- Toxicity to aquatic algae and cyanobacteria
- Toxicity to aquatic plants other than algae
- Toxicity to microorganisms
- Endocrine disrupter testing in aquatic vertebrates – in vivo
- Toxicity to other aquatic organisms
- Sediment toxicity
- Terrestrial toxicity
- Biological effects monitoring
- Biotransformation and kinetics
- Additional ecotoxological information
- Toxicological Summary
- Toxicokinetics, metabolism and distribution
- Acute Toxicity
- Irritation / corrosion
- Sensitisation
- Repeated dose toxicity
- Genetic toxicity
- Carcinogenicity
- Toxicity to reproduction
- Specific investigations
- Exposure related observations in humans
- Toxic effects on livestock and pets
- Additional toxicological data
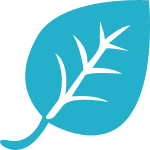
Phototransformation in water
Administrative data
Link to relevant study record(s)
- Endpoint:
- phototransformation in water
- Type of information:
- experimental study
- Adequacy of study:
- key study
- Study period:
- 1995-12-08 - 1996-04-24
- Reliability:
- 1 (reliable without restriction)
- Rationale for reliability incl. deficiencies:
- guideline study
- Qualifier:
- according to guideline
- Guideline:
- EPA Guideline Subdivision N 161-2 (Photodegradation Studies in Water)
- Deviations:
- no
- GLP compliance:
- yes
- Remarks:
- U.S. EPA Good Laboratory Practice Standards (40 CFR Part 160)
- Specific details on test material used for the study:
- Purity analyses were performed by proton nuclear magnetic resonance spectroscopy (NMR) on the actual [14C]ferbam test substance used for dosing.
- Radiolabelling:
- yes
- Analytical method:
- high-performance liquid chromatography
- other: Radioassay, Thin Layer Chromatography
- Details on sampling:
- Sampling
Duplicate sample tubes were removed from the irradiated and dark control sets following 1, 2, 3, 7 and 15 days (based on calendar dates rather than 24-hour time periods). One set of tubes (constructed from quartz) was irradiated in duplicate (two for analysis at 5 discrete time intervals). The other set (dark control, constructed from Pyrex®) was wrapped in aluminum foil to prevent irradiation. Dark controls were placed in the same water bath with the irradiated sample tubes but were outside the irradiation chamber. At each sampling interval, appropriate samples were removed from the photolysis chamber. The test solution was transferred to a foil-covered, silanized glass vial, 1 ml removed for pH determination with a Corning Model 240 pH meter (Corning Science Products, Corning, NY), and the remainder partitioned immediately with an equal volume of chloroform to inhibit further hydrolytic degradation (3 x 0.5-mI aliquots were taken prior to partitioning on Day 1). Aliquots (3 x 100 uL) of the aqueous and chloroform phases were taken immediately, for radioassay. The pH values were recorded at each sampling interval. Chloroform and aqueous phases were subsequently analyzed by HPLC, then stored under freezer conditions (approximately -20°C). Each photolysis tube, following removal of the test solution, was rinsed with 2.0 ml of acetonitrile to remove the residual aqueous photolysis solution and any material bound to the tube walls. These rinses were radioassayed (3 x 100 uL) and analyzed separately by HPLC.
Ion-Pair Partitioning of the Aqueous Partition Phase of Day 7. Irradiated. Replicate B
In an effort to further characterize components of the aqueous partition phase, a 1-ml aliquot of the Day 7, Irradiated, Replicate B sample was amended with an ion-pair reagent (0.25 ml of tetrabutylammonium phosphate) and partitioned with 1.2 ml of chloroform. The chloroform phase was subsequently amended with 3.1 fil of iodomethane, a methylating reagent, and the mixture allowed to react for 30 minutes at room temperature. HPLC was performed on both the aqueous and chloroform phases following ion-pair partitioning.
Trap Solution Maintenance and Sampling
Trap solutions were changed periodically and at each sampling interval during the study. Fresh traps were installed containing either 50 or 70 ml of methanolic KOH, 50 ml of ethanol and either 30 or 50 ml of ethylene glycol. Since the initial methanolic KOH trap decreased in volume during continuous flushing with condensation of the methanol primarily in the ethylene glycol trap, volumes of the methanolic KOH and ethylene glycol traps were adjusted to either accommodate the time between sample intervals or to maximize the time between trap changes.
Trapped radiocarbon was found primarily in the methanolic KOH solutions with the initial trap containing most of the radiocarbon. In addition to radiocarbon trapped in solution, radiocarbon was also found in the glass frit of the methanolic KOH trap dispersion tube as precipitated salt. After removal of the methanolic KOH solution, the dispersion tube frit was rinsed with 15 ml of DIUF water by drawing water into the frit and evacuating several times then sonicating for approximately 5 minutes. The methanolic KOH trap solutions and water rinses were radioassayed and retained separately. Precipitation of the methanolic KOH trap solutions and water rinses with barium chloride was performed following dilution (1:1, v:v) with DIUF water or methanolic KOH, respectively. - Buffers:
- not specified
- Light source:
- Xenon lamp
- Light spectrum: wavelength in nm:
- >= 250 - <= 800
- Details on light source:
- Intensity of the xenon lamps was measured using an International Light Radiometer (Model IL 1700, International Light, Inc., Newburyport, MA) equipped with a Model SUD 400 photodetector enclosed in a quartz casing (1/8" thick) located within the irradiation chamber and submerged in the water bath. Intensity measurements were monitored at intervals of 10 seconds and recorded at 10-minute intervals with the Campbell Scientific Model 2IX Datalogger. Intensity measurements were used to confirm the output of the xenon lamps during the course of the study and to allow calculation of a total intensity for the study period.
The spectral distribution of emitted light from the xenon lamps was determined at the beginning and end of the study to assess any effects of lamp aging during the course of the study. Spectral intensity was measured at each 10-nm increment from 250-800 nm using an IL 782 monochromator/photomultiplier, an IL 760 D photomultiplier power supply and an EL 1700 radiometer. These instruments were obtained from International Light, Inc. (Newburyport, MA). These measurements present monochromatic intensity at each specified wavelength as opposed to the integrated intensity in the 10-nm window. - Details on test conditions:
- Non-radiolabelled reference substances for tetramethylthiuram disulfide (TMTD) and l,l,3,3-tetramethyl-2-thiourea (TMTU) were obtained from Aldrich Chemical Company (Milwaukee, WT). Tetramethylthiuram monosulfide (TMTM) was obtained from Lancaster Synthesis, Inc. (Windham, NH). Methylisothiocyanate (MITC) was obtained from Crescent Chemical Co., Inc. (Hauppauge, NY). Carbon disulfide (CS2), 14CS2 and 14C02 (sodium bicarbonate salt) were obtained from Sigma Chemical Company (St. Louis, MO). Non-radiolabelled standards of TMTD, TMTM, TMTU and MITC, and CS2 were analyzed by HPLC (qualitatively) prior to use in the study and following the last sampling to confirm stability.
Solvents used were HPLC-grade or higher quality and were obtained from Fisher Scientific (Pittsburgh, PA). Deionized ultrafiltered (DIUF) water was also obtained from Fisher Scientific. Trypticase soy agar and potato dextrose agar were obtained from Difco Laboratories (Detroit, MI). Safety-Solve™ scintillation cocktail (Research Products International, Mount Prospect, IL) was used for all liquid scintillation counting (LSC) and 14C-radiocarbon detection by high performance liquid chromatography (HPLC). Iodomethane was obtained from Aldrich Chemical Company. Tetrabutylammonium phosphate was obtained from Eastman Kodak Co., Rochester, NY. All other chemicals used were reagent-grade or higher quality.
Apparatus
All photolysis glassware was autoclaved prior to use (121 °C, 15 psi, approximately 30 minutes). Tubes for irradiated solutions were constructed from quartz (11-mm internal diameter x 205-mm length). Dark control tubes were constructed from Pyrex® and foil-covered. Each set of tubes (irradiated and dark controls) were continuously purged with humidified air under negative pressure with the air being passed through a series of volatile collection traps. Ambient air was drawn through a Gelman bacterial air vent (Model No. 4210, Gelman Sciences, Ann Arbor, MI) at approximately 50 to 70 ml per minute, then bubbled through a humidifying flask containing filter-sterilized DIUF water. The humidified air was continuously drawn through the test system into a series of foil-covered traps (dispersion tubes) containing methanolic IN KOH (two traps), ethanol and ethylene glycol for collection of volatile compounds. A separate set of traps was used for the irradiated and dark control sample sets. Traps were filled with 50 ml of trapping solution at study initiation.
The quartz tubes (immersed in a -13" x 84" tank containing approximately 6" of deionized water) were irradiated in a chamber designed and developed for the exposure of test samples to xenon light. The photolysis source was two xenon lamps (1500 W each) filtered with a borosilicate glass cut off sleeve for removal of light with wavelengths less than 290 nm. Dark control tubes were immersed in the same water bath outside the irradiation chamber. It is well established that no attenuation of direct sunlight occurs by its passage through a 6" depth of water (Reference 2). Tubes were oriented at a 30° angle with respect to the horizontal. The temperature of the water bath was maintained at a mean temperature of 24.9 ± 0.1 °C (mean ± standard error) during the study by circulating the water through a Lauda Constant Temperature Circulator (Model RMT-20 Brinkman Instruments, Inc., Westbury, NY). Temperatures were monitored at intervals of 10 seconds, then averaged and recorded at 10-minute intervals throughout the study using a Campbell Scientific Model 21X Datalogger and a thermistor-type Model 107B temperature probe (Campbell Scientific, Inc., Logan, UT).
Preparation of Test Substance and Dosing of the Test System
Solutions of [14C]ferbam (specific activity of 59.96 mCi/mmole, 144.14 uCi/mg) were prepared in chloroform-d3 and assayed for identity and purity by NMR. Subsequently, the contents of one NMR tube (990-20, originally designated for the photolysis study) was transferred to a sterile 500-ml, silanized, Erlenmeyer flask using aseptic technique. The chloroform was allowed to evaporate. A solution of [13C]ferbam, (990-14) previously assayed for purity by NMR, was prepared in acetonitrile at a concentration of 1 mg/ml. [13C]Ferbam, 1.6 mg (1.6 ml), was added to the flask to achieve a final nominal concentration of 10 ppm for a total volume of 220 ml of test solution based on the anticipated amount of [14C]ferbam present. Radioassay showed the solution concentration to be lower in [14C]ferbam than expected based on radiocarbon originally in the NMR tube. An aliquot of [14C]ferbam from a second NMR tube (990-24) was allowed to dry under nitrogen, resolubilized in 50 ul of acetonitrile and added to the test solution. An additional 50 uL of acetonitrile was added to achieve a final cosolvent concentration of 1 % by volume. Radioassay of the test solution confirmed the presence of adequate radiocarbon for subsequent analytical monitoring. Aliquots of the test solution, 10 ml each, were transferred first to ten quartz tubes (synonyms light exposed, LE and irradiated) and then to ten Pyrex® tubes (dark controls). The concentration of [14C]ferbam was determined from radioassay of aliquots of the test solution taken prior to dosing (81,052 dpm/100 uL), following dosing of the quartz tubes (82,072 dpm/100 uL) and following dosing of the Pyrex® tubes (83,245 dpm/100 uL). The average concentration of [14C]ferbam was 82,123 dpm/100 uL, equivalent to 2.6 [ug/ml which added to the [13C]ferbam concentration of 7.3 ug/ml gave a final concentration of 9.9 ppm.
Test System and Sample Identification
The test system consisted of photolysis tubes containing [13C/,4C]ferbam in sterile deionized ultra-filtered water. Each photolysis tube was identified by PTRL East, Inc. project number, ,4C sample identification, personnel initials and date of 14C application.
Sterility Check of Test Solution
On Day 0 and Day 15, 1.0-ml aliquots were removed from the test solution (four aliquots from single Day 0 subsample and two aliquots from the Day 15, Replicate A and B, irradiated and dark control tubes) to confirm the sterility of the test solution. Aliquots of the test solution were plated in trypticase soy agar (TSA) and potato dextrose agar (PDA). The TSA plates were incubated for one day at 35°C . The PDA plates were incubated for two days at 25°C. The test system remained sterile throughout the study period.
CALCULATIONS
Calculations were performed using the following formulas:
Note: Dpm values from LSC sheets are truncated to the whole number for all calculations. Detection limit for all samples is twice background.
A. Radiocarbon Present in Solution at Sampling Time
((Raw dpm - background dpm) / Partition factor) * ((Volume (mL) of solution in tube) / Aliquot volume)
B. Total DPM Recovered in Solution per Tube
Dpm in organic phase + Dpm in aqueous phase = Dpm recovered/tube
C. Methanolic KOH. Ethanol and Ethylene Glycol Trap Solutions
(Raw dpm - background dpm) x (Total volume / Aliquot volume) = (Total dpm in trap solution / Number of dishes in chamber) = Trapped radiocarbon attributed to each soil dish
D. Total Percent Recovery in Solution per Tube
((Total dpm recovered in solution/tube) / (total dpm applied/tube)) x 100 = Percent total recovery
E. Quantitative Characterization
(Percent of radiocarbon analyzed by HPLC as a gien compound) x (Percent of applied radiocarbon in solution) / 100 = Percent of applied radiocarbon
F. Half-Life
The photolysis rate constant and half-life of ferbam were calculated using pseudo first-order reaction kinetics. Assuming first-order degradation, the degradation rate constants were calculated from the following equations:
ln C = -k t + ln C0 (y = mx + b)
Where: k = rate constant
C = chemical concentration at time t
t = time
C0 = initial chemical concentration (t = 0)
The half-life of ferbam was calculated using the following equation:
t1/2 = ln 2 / k
HPLC Recovery
Aliquots of solutions prepared for HPLC injection were radioassayed in triplicate before injection. After injection, the HPLC eluate was collected (total run) and radioassayed in triplicate.
% Recovery = ((Eluate aliquot dpm - Background) x (Eluate volume / Aliquot volume*)) / ((Sample aliquot volume - Background) x (Injection volume / Aliquot volume**)) x 100
* Aliquot volume of radioassayed eluate
** Aliquot volume of radioassayed injection solution
Statistical Methods
Means, standard deviations, standard errors and linear regression were the only statistical analyses performed on the data. - Duration:
- 15 d
- Temp.:
- 24.9 °C
- Initial conc. measured:
- 9.9 other: ppm
- Reference substance:
- not specified
- Dark controls:
- yes
- Computational methods:
- Analysis of Radiochromatography Data
Prior to integrating each radiochromatogram, a five-point smoothing routine available in the software was used to improve peak detection. The integration parameters for peak identification were as follows:
Parameter: System Setting:
+ peak threshold + 0.600
- peak threshold - 0.600
minimum peak area 50
bachground set by run (detailed below)
filter 13
baseline no baseline
Background radioactivity for each set of analyses run on the instrument was determined by integrating a period of the chromatogram (ca. 5 minutes) containing no peaks then calculating the background.
The background radioactivity in cpm was entered as a peak integration parameter for each set of analyses. The backgrounds obtained averaged 135 with a standard deviation of 43 cpm. HPLC column recovery was determined for each chromatographic run by radioassay of triplicate aliquots of the injection solution compared to the radiocarbon content of the total HPLC column eluate collected for each run (see Calculations)
Radioactive compounds separated by TLC were quantitatively analyzed by removing zones from the plate, solubilizing each zone in methanol for approximately one hour, adding cocktail and radioassaying by liquid scintillation counting. The dpm in each zone were expressed as a percentage of the total dpm recovered. Recovery of radiocarbon from TLC plates was determined by comparison of the amount of applied radiocarbon to the sum of radiocarbon recovered from scrapings of each sample lane. - Preliminary study:
- not specified
- Test performance:
- not specified
- Remarks on result:
- other: see 'Details on results'
- Remarks on result:
- other: see 'Details on results'
- Predicted environmental photolytic half-life:
- not specified
- Transformation products:
- yes
- Details on results:
- Ferbam has a characteristic reverse-phase chromatographic profile containing multiple peaks with leading fronts and tails indicative of on-column degradation. The major on-column degradation products that appear as discrete peaks cochromatograph with tetramethylthiuram disulfide (TMTD) and CS2. In addition, the chromatogram of ferbam shows a characteristic broad region of radiocarbon eluting prior to TMTD or CS2. TMTD, formed as a result of degradation prior to column injection, can be distinguished from ferbam chromatographically since TMTD chromatographs as a distinct peak well resolved from the broad early-eluting region characteristic of ferbam on-column degradation.
Justification of the Test System
The relatively rapid hydrolysis of metal dithiocarbamates such as ferbam in acidic and basic aqueous solutions forms the basis of methods for determination of total residue via conversion to CS2 and DTC anions, respectively. Since hydrolysis is rapid at pH 5, 7 and 9, DIJJF water was selected as the test medium for study of the photolysis to preclude reactivity of degradation products, e.g., DTC anions, with buffer salts. A continuous irradiation regime was used to maximize photolytic degradation relative to hydrolysis.
Purity of [14C]Ferbam
As a result of the instability of ferbam in either reverse- or normal-phase HPLC or TLC chromatographic systems, purity of [14C]ferbam and [13C]ferbam were established by NMR spectroscopy prior to application of the blended test substance to solution. The actual contents of NMR tubes containing [14C]ferbam were subsequently used for preparation of the dosing solution. Non-radiolabelled reference substances were analyzed qualitatively by HPLC prior to initiation of the study and following the last sampling interval for determination of stability.
Exposure of Test Solution to Xenon Light
Irradiated and dark control samples were placed in the water bath (irradiated samples under xenon lamps 24 hours per day) for 15 days (February 26, 1996 - March 12, 1996), equivalent to a 30-day natural sunlight exposure. Duplicate samples were removed for analysis. Temperatures and total irradiation intensity data recorded throughout the study. The water bath temperature was 24.9 ± 0.1 °C (mean ± standard error). The average light intensity per day for the entire study was 5,554 ± 31 uW/cm2 (mean ± standard error). Light energy, expressed as Wmin/cm2/day, was 8.0 ± 0.0 Wmin/cm2/day (mean ± standard error). For the 15-day study, cumulative light energy was 120.1 Wmin/cm2.
Material Balance of i14ClFerbam Throughout the Study Period
Total radiocarbon recoveries (mean ± standard deviation) for irradiated and dark control samples were 95.3 ± 1.6% and 100.7 ± 1.7%, respectively.
pH Values Throughout the Study Period
The pH of test solutions became more acidic during the study period decreasing from 6.03 to 5.15 over the 15-day incubation period (mean of irradiated and dark control replicates). This could potentially indicate the formation of acidic products. However, it is noted that the ionic strength of these solutions, prepared with deionized ultrafiltered water, may be too low for reliable determination of pH.
Degradation of [14C]Ferbam
Hydrolysis was too rapid to permit assessment of the photolytic degradation of ferbam per se. An experiment, performed during the course of the study of hydrolysis of ferbam (PTRL East, Inc. Project No. 990), yielded the most unequivocal results regarding degradation rate. Results of this experiment, performed at concentration of 10 ppm, showed a maximum hydrolysis half-life of ferbam in DIUF water of 31.0 minutes. Although photolysis of ferbam per se could not be studied, photolytic degradation of ferbam and hydrolysis products was possible. The most notable difference in degradation between irradiated and dark control samples was the rate and extent of formation of volatile products. Following 15 days of incubation, volatile products accounted for 73.6% of the applied radiocarbon for irradiated samples compared to 31.4% for samples maintained in darkness. Quantitative assessment of the photolytic contribution to degradation was complicated by the absence of either first-order degradation of total applied radiocarbon to volatile products or first-order degradation of the primary product remaining in solution, TMTD. In the dark control, degradation to volatile products was rapid through Day 2 but slowed thereafter whereas for irradiated samples, degradation to volatile products continued. There was, however, reasonably good correlation of the difference in TMTD concentrations, between irradiated and dark control samples, with first-order kinetics for samples collected through 7 days. Day 15 samples were excluded since the concentration of TMTD was near non-detectable levels at both 7 and 15 days for irradiated samples (1.1 and 0.5% of the applied radiocarbon, respectively). The half-life attributed to photolytic degradation of the primary product, TMTD, was approximately 4.7 days (r2 = 0.9653).
Quantitative Characterization of [14C]Ferbam and Its Degradates
Ferbam degraded rapidly in aqueous media with formation of TMTD and volatile products. In irradiated samples, TMTD further degraded to volatile products, found primarily in trap solutions, and polar products, found mainly in the aqueous phase following partitioning of incubated solutions with chloroform. Following one day of incubation, there was no chromatographic indication of the presence of ferbam in incubated solutions, i.e., no broad early-eluting region on HPLC or extraneous peaks characteristic of ferbam on-column degradation in the chloroform partition phase.
Degradation to volatile products was significant under both irradiated and dark control conditions but considerably greater under irradiated conditions. Following one day of incubation, trapped volatiles accounted for 13.8% of the applied radiocarbon for irradiated samples and 9.0% for dark controls. Following 15 days of exposure, volatile products accounted for 73.6% of the applied radiocarbon for irradiated samples versus 31.4% for dark controls (Table VI). Barium chloride precipitation of the methanolic KOH trap solutions and water rinses of the traps indicated that both CS2 and CO2 were present. Following 15 days of irradiation, CS2 in the traps accounted for 44.6% of the applied radiocarbon versus 28.6% CO2. For dark control solutions, CS2 accounted for 27.3% of the volatile radiocarbon versus 3.5% CO2.
In addition to CS2 in trap solutions, CS2 was also found in the organic partition phase (chloroform) of incubated solutions and, to a lesser extent, in acetonitrile tube rinses following removal of incubated solutions. Maximum amounts in the organic partition phase and tube rinses of solutions were 6.8% of the applied radiocarbon at Day 3 for irradiated samples (mean of replicates) and 6.1% at Day 2 for dark controls (mean of replicates). CS2 retained in solution and tube rinses decreased significantly at later sample intervals to 0.2 and 0.4% of the applied radiocarbon for irradiated and dark control samples at Day 15, respectively.
Radiocarbon remaining in solution at Day 1 for both irradiated and dark control solutions was found predominantly in the organic (chloroform) partition phase. Following 15 days of incubation, radiocarbon remaining in solution of irradiated samples was predominantly in the aqueous partition phase versus the organic phase for dark control samples.
Acetonitrile rinses of the tubes following removal of incubated solutions showed varying levels of radiocarbon remaining in the tubes. In general, the amounts were consistent between irradiated and dark control samples and decreased with incubation time. The mean radiocarbon concentration at Day 1 in tube rinses for both irradiated and dark control samples was 6.7% with a range of 2.4 - 11.5%. Following 15 days of incubation, the mean radiocarbon concentration was 1.6% with a range of 0.4 - 2.7%.
TMTD was the primary non-volatile degradation product accounting for 63.3 and 73.1% of the applied radiocarbon for irradiated and dark control samples, respectively, at Day 1. TMTD concentrations remained relatively constant in dark control samples decreasing to 61.1% of the applied radiocarbon following 15 days of incubation. In contrast, TMTD concentrations decreased to 1.1 % by Day 7 and 0.5% of the applied radiocarbon by Day 15 in irradiated samples. TMTD was found primarily in the organic partition phase of incubated samples with significantly lesser amounts found in acetonitrile tube rinses.
For irradiated samples, two additional products were formed exceeding 10% of the applied radiocarbon, designated Degradates A and B. No other products, other than TMTD and volatile products, were observed exceeding 10% of the applied radiocarbon. Degrádate A was found in both irradiated and dark control samples. Degrádate A increased in concentration for irradiated samples but remained relatively constant for dark controls. The product reached a maximum of 16.0% (mean of replicates) in irradiated samples following 3 days of irradiation and decreased to 9.0% by Day 15. In the dark controls, this product was approximately 5.6% of the applied radiocarbon throughout the incubation period. Degrádate B was found only in trace amounts in the dark control. In irradiated samples, Degrádate B was not detected at Day 1 but increased to 11.9% (mean of replicates) following 15 days of irradiation. Degrádate A was found predominantly in the aqueous partition phase with lesser amounts in the acetonitrile tube rinses. Degrádate B was found in both organic and aqueous partition phases.
In an effort to further characterize aqueous phase components (Degradates A and B), an aliquot of the aqueous partition phase of Day 7, Irradiated, Replicate B was amended with an ion-pair reagent (tetrabutylammonium phosphate, TBAP). The mixture was then partitioned with chloroform. Components of the chloroform phase were reacted with iodomethane, a methylating reagent. TBAP was effective as an ion-pair reagent and allowed partitioning of 56.6% of the radiocarbon from the aqueous solution. HPLC of the aqueous phase following ion-pair partitioning showed multiple components not observed in the sample prior to partitioning. HPLC of the chloroform phase showed peaks at essentially the same retention times as Degradates A and B. Extractability of Degradates A and B by TBAP showed both to be acidic in nature. Further, the presence of multiple peaks in the aqueous phase following partitioning showed possible reactivity of Degradates A and B with TBAP. The presence of Degradates A and B in the HPLC of the chloroform phase indicated that iodomethane was not effective in methylating these components.
In summary, ferbam and its degradation products, arising from hydrolysis, degraded more rapidly under irradiated than dark conditions. Primary degradation products under irradiated conditions were volatile, primarily CS2. Radiocarbon remaining in solution also showed significantly more degradation under irradiated than dark conditions. TMTD was the major product found in dark control solutions accounting for 73.1 to 61.1% of the applied radiocarbon from Day 1 through Day 15. In irradiated solutions, TMTD was the major degrádate following one day of irradiation, 63.3% of the applied radiocarbon, and decreased to 1.1% at 7 days. Degrádate A, determined by HPLC, was present following one day of irradiation (7.7% of the applied radiocarbon), increased to a maximum of 16.0% following 3 days, and subsequently decreased to 9.0% following 15 days of irradiation. Degrádate B, found only to a significant extent in irradiated solutions, increased from 0.0% to a maximum of 11.9% at 15 days. Degradates A and B were characterized as polar, water soluble and acidic in nature. Degrádate profiles for irradiated and dark control samples are presented in Figures 18 and 19, respectively. It cannot be determined from the profile for the irradiated samples whether TMTD degrades directly to CS2 and CO2 or through intermediates. It does appear that Degrádate B arises from degradation of Degrádate A.
Two one-dimensional TLC systems were used to confirm the HPLC characterization of TMTD in the organic partition phase of Day 1 and Day 3, Replicates A and B, irradiated samples and in the Day 15, Replicate A and B, dark controls. Plate recoveries, obtained by summing the radiocarbon in all zones of the TLC plates, were <90% for irradiated samples (79.4 - 85.7%). The loss of radiocarbon was attributed to the volatility of TMTD. Thus, the quantities of degradation products relative to TMTD may be overstated. TLC confirmed the presence of TMTD as the major component in both irradiated and dark control samples. Additional degradates were present in both irradiated and dark control samples although more prevalent in irradiated samples. Other than TMTD, origin-bound material was the most prevalent region of radiocarbon. Origin-bound material accounted for 2.5 and 8.6% of plate recovered radiocarbon for Day 1 and Day 3 irradiated samples (mean of replicates) in the toluene:methanol system and 2.2 and 2.9% in the chloroform:acetonitrile system. Although TMTU was not observed by HPLC, a region of radiocarbon cochromatographed with TMTU in both systems for irradiated samples. TMTU concentrations averaged 0.5 and 3.5% of plate recovered radiocarbon for Day 1 and Day 3 irradiated samples (mean of replicates) in the to!uene:methanol system and 4.9 and 3.5% in the chloroform:acetonitrile system. Minor unknown degradates were also observed by TLC for irradiated and dark control samples, < 6.5 and < 2.4% of plate recovered radiocarbon for a single degradate, respectively. However, none of the degradates cochromatographed in both systems for dark controls with either TMTM or TMTU.
Sample Storage Stability
Extraction of photolysis solutions with chloroform was performed immediately following sample collection. HPLC analyses were performed as soon as possible after sample collection, typically within 48 hours of collection. Samples were maintained under freezer conditions (-20°C) for any interim storage. Samples (representative of tube rinses, aqueous partition phases and organic partition phases), reanalyzed by HPLC following two to four weeks of storage, did not show appreciable changes in chromatographic profiles versus the initial analyses thus confirming stability of samples in storage. - Results with reference substance:
- not specified
- Conclusions:
- [14C]Ferbam in aqueous buffered solution (deionized ultrafiltered water) at a nominal 10-ppm concentration was irradiated for 15 days in artificial light. Solutions maintained under the same conditions except in darkness served as controls. Hydrolysis of ferbam was too rapid to permit meaningful assessment of photolysis of ferbam per se with the degradation half-life in DIUF water estimated to be <31.0 minutes. However, photolysis of ferbam and its hydrolysis products was found to be significant. Ferbam degraded initially to tetramethylthiuram disulfide. The terminal photolysis product was predominantly CS2. The absence of first-order degradation precluded determination of a quantitative measure of the contribution of photolysis to overall degradation. Photolysis however would contribute significantly to overall dissipation of ferbam in aqueous environments.
- Executive summary:
This study was designed and conducted according to the U.S. EPA Pesticide Assessment Guidelines, Subdivision N, Series 161-2, to establish the significance of solution photolysis in artificial light (xenon) as a route of degradation for [14C]ferbam and to quantitate any degradation products formed.
Ferbam was sufficiently unstable in either reverse- or normal-phase high performance liquid chromatography (HPLC) or thin layer chromatography (TLC) to preclude these methods for determination of purity of the test substance. As a result of this instability, the purity of [14C]ferbam was determined in a chloroform-d3 solution by proton nuclear magnetic resonance spectroscopy (NMR). Purity of [13C]ferbam in acetonitrile-d3 solution was likewise determined by NMR. Just prior to dosing, aliquots of [14C]ferbam and [13C]ferbam solutions were transferred aseptically to a sterile, autoclaved Erlenmeyer flask. The organic solvent was removed with a gentle stream of nitrogen. Acetonitrile was added to achieve a 1 % cosolvent level to assist solubilization of ferbam in the aqueous solution. Sterile deionized ultra-filtered (DIUF) water was then added to achieve a concentration of 9.9 ppm. A subsample of this mixture was designated as Day 0 and sampled immediately following dosing. The remaining mixture was placed in either quartz (irradiated) or Pyrex® (dark controls) tubes. Four tubes were designated for each sampling interval (two irradiated samples and two dark control samples). Dark control tubes were wrapped in aluminum foil to prevent irradiation. Each set of tubes (irradiated and dark controls) were continuously purged with air under negative pressure with the air being passed through a series of volatile collection traps. Traps consisted of two methanolic KOH traps (1N), one ethanol trap and one ethylene glycol trap in series. During the study period, samples were maintained at a temperature of 24.9 ± 0.1°C (mean ± standard error). Samples were collected following 1, 2, 3, 7 and 15 days of incubation. Quantitative analyses of samples were determined by reverse-phase high performance liquid chromatography.
Instability of ferbam at low concentrations in aqueous solution precluded assessment of photolysis of ferbam per se. The degradation half-life in DIUF water was estimated to be < 31.0 minutes. Photolysis did however contribute significantly to degradation of hydrolysis products. Considerably greater formation of volatile products, CS2 and CO2, was observed for irradiated solutions versus dark controls. Following 15 days of continuous irradiation, total volatile formation for irradiated samples amounted to 73.6% of the applied radiocarbon versus 31.4% for dark controls. CS2 was the primary component of volatile products (44.6% versus 28.6% CO2) in D15 irradiated samples. The primary product remaining in solution for both irradiated and dark control samples cochromatographed with tetramethylthiuram disulfide (TMTD). This product was also photolytically unstable degrading primarily to volatile products. Two additional degradation products >10% of the applied radiocarbon were observed in irradiated solutions. These products were water soluble and acidic in nature. Photolysis of the hydrolysis product mixture resulting from ferbam degradation did not follow first-order degradation.
Total radiocarbon recovery (mean ± standard deviation) for irradiated samples was 95.3 ± 1.6%. For dark control samples, radiocarbon recovery from the test system was 100.7 ± 1.7%.
Reference
Description of key information
According GLP and U.S. EPA Pesticide Assessment Guidelines, Subdivision N, Series 161 -2 [14C]Ferbam in aqueous buffered solution (deionized ultrafiltered water) at a nominal 10-ppm concentration was irradiated for 15 days in artificial light. Solutions maintained under the same conditions except in darkness served as controls. Hydrolysis of ferbam was too rapid to permit meaningful assessment of photolysis of ferbam per se with the degradation half-life in DIUF water estimated to be <31.0 minutes. However, photolysis of ferbam and its hydrolysis products was found to be significant. Ferbam degraded initially to tetramethylthiuram disulfide. The terminal photolysis product was predominantly CS2. The absence of first-order degradation precluded determination of a quantitative measure of the contribution of photolysis to overall degradation. Photolysis however would contribute significantly to overall dissipation of ferbam in aqueous environments.
Key value for chemical safety assessment
Additional information
Key: Nixon, 1996
This study was designed and conducted according to the U.S. EPA Pesticide Assessment Guidelines, Subdivision N, Series 161-2, to establish the significance of solution photolysis in artificial light (xenon) as a route of degradation for [14C]ferbam and to quantitate any degradation products formed.
Ferbam was sufficiently unstable in either reverse- or normal-phase high performance liquid chromatography (HPLC) or thin layer chromatography (TLC) to preclude these methods for determination of purity of the test substance. As a result of this instability, the purity of [14C]ferbam was determined in a chloroform-d3 solution by proton nuclear magnetic resonance spectroscopy (NMR). Purity of [13C]ferbam in acetonitrile-d3 solution was likewise determined by NMR. Just prior to dosing, aliquots of [14C]ferbam and [13C]ferbam solutions were transferred aseptically to a sterile, autoclaved Erlenmeyer flask. The organic solvent was removed with a gentle stream of nitrogen. Acetonitrile was added to achieve a 1 % cosolvent level to assist solubilization of ferbam in the aqueous solution. Sterile deionized ultra-filtered (DIUF) water was then added to achieve a concentration of 9.9 ppm. A subsample of this mixture was designated as Day 0 and sampled immediately following dosing. The remaining mixture was placed in either quartz (irradiated) or Pyrex® (dark controls) tubes. Four tubes were designated for each sampling interval (two irradiated samples and two dark control samples). Dark control tubes were wrapped in aluminum foil to prevent irradiation. Each set of tubes (irradiated and dark controls) were continuously purged with air under negative pressure with the air being passed through a series of volatile collection traps. Traps consisted of two methanolic KOH traps (1N), one ethanol trap and one ethylene glycol trap in series. During the study period, samples were maintained at a temperature of 24.9 ± 0.1°C (mean ± standard error). Samples were collected following 1, 2, 3, 7 and 15 days of incubation. Quantitative analyses of samples were determined by reverse-phase high performance liquid chromatography.
Instability of ferbam at low concentrations in aqueous solution precluded assessment of photolysis of ferbam per se. The degradation half-life in DIUF water was estimated to be < 31.0 minutes. Photolysis did however contribute significantly to degradation of hydrolysis products. Considerably greater formation of volatile products, CS2 and CO2, was observed for irradiated solutions versus dark controls. Following 15 days of continuous irradiation, total volatile formation for irradiated samples amounted to 73.6% of the applied radiocarbon versus 31.4% for dark controls. CS2 was the primary component of volatile products (44.6% versus 28.6% CO2) in D15 irradiated samples. The primary product remaining in solution for both irradiated and dark control samples cochromatographed with tetramethylthiuram disulfide (TMTD). This product was also photolytically unstable degrading primarily to volatile products. Two additional degradation products >10% of the applied radiocarbon were observed in irradiated solutions. These products were water soluble and acidic in nature. Photolysis of the hydrolysis product mixture resulting from ferbam degradation did not follow first-order degradation.
Total radiocarbon recovery (mean ± standard deviation) for irradiated samples was 95.3 ± 1.6%. For dark control samples, radiocarbon recovery from the test system was 100.7 ± 1.7%.
Supporting Information: Warren, 1986
A photolysis study was conducted according to EPA Guideline Subdivision N 161-2 (Photodegradation Studies in Water) with 14C-Ferbam in sterile deionized water and in sterile deionized water containing 1% acetone as a sensitizing agent. Light and dark sensitized and unsensitized test systems were conducted. The light test system were exposed to a Xenon Arc Light source. Quantification of total 14C-Ferbam residues in the test systems was measured by liquid scintillation counting (LSC) analysis. Characterization and quantification of Ferbam was by spectrophotometry analysis.
The photolysis rate and half-life of 14C-Ferbam in the light and dark unsensitized test system was calculated based on spectrophotometric data obtained. The photolysis rate of 14C-Ferbam in the light unsensitized test system was determined to be -0.03605 with a half-life of 19 hours. The photolysis rate of 14C-Ferbam in the dark unsensitized test system was determined to be -0.05601 with a half-life of 12 hours.
Comparison of the light and dark unsensitized systems indicated that 14C-Ferbam degrades rapidly in aqueous solutions regardless of its exposure to light. Therefore, the degradation of 14C-Ferbam in aqueous solution does not appear to be a photosensitized reaction.
The photolysis rate and half-life of 14C-Ferbam in the sensitized test system could not be calculated, due to the variability in spectrophotometric data obtained.
An approximate degradation rate and half-life of 14C-Ferbam in the sensitized systems was determined from the LSC data. A degradation rate calculated based on the degradation of total 14C-residues of Ferbam in the test systems. The degradation rates of the 14C-residues of Ferbam in the sensitized light test system was -0.02933 with a half-life of 24 hours. The degradation rate of the 14C-residues of Ferbam in the sensitized dark test system was -0.01331 with a half-life of 52 hours.
A duplicate set of light and dark, sensitized and unsensitized test systems were also conducted. This second set of test systems was conducted specifically to monitor the evolution of 14C-volatiles and to determine 14C-mass accountability. The 14C-mass accountability for the light and dark unsensitized test systems was 95.8% and 101.2%, respectively. The 14C-mass accountability for the light and dark sensitized test systems was 93.1 and 101.4%, respectively.
Information on Registered Substances comes from registration dossiers which have been assigned a registration number. The assignment of a registration number does however not guarantee that the information in the dossier is correct or that the dossier is compliant with Regulation (EC) No 1907/2006 (the REACH Regulation). This information has not been reviewed or verified by the Agency or any other authority. The content is subject to change without prior notice.
Reproduction or further distribution of this information may be subject to copyright protection. Use of the information without obtaining the permission from the owner(s) of the respective information might violate the rights of the owner.
