Registration Dossier
Registration Dossier
Data platform availability banner - registered substances factsheets
Please be aware that this old REACH registration data factsheet is no longer maintained; it remains frozen as of 19th May 2023.
The new ECHA CHEM database has been released by ECHA, and it now contains all REACH registration data. There are more details on the transition of ECHA's published data to ECHA CHEM here.
Diss Factsheets
Use of this information is subject to copyright laws and may require the permission of the owner of the information, as described in the ECHA Legal Notice.
EC number: 200-817-4 | CAS number: 74-87-3
- Life Cycle description
- Uses advised against
- Endpoint summary
- Appearance / physical state / colour
- Melting point / freezing point
- Boiling point
- Density
- Particle size distribution (Granulometry)
- Vapour pressure
- Partition coefficient
- Water solubility
- Solubility in organic solvents / fat solubility
- Surface tension
- Flash point
- Auto flammability
- Flammability
- Explosiveness
- Oxidising properties
- Oxidation reduction potential
- Stability in organic solvents and identity of relevant degradation products
- Storage stability and reactivity towards container material
- Stability: thermal, sunlight, metals
- pH
- Dissociation constant
- Viscosity
- Additional physico-chemical information
- Additional physico-chemical properties of nanomaterials
- Nanomaterial agglomeration / aggregation
- Nanomaterial crystalline phase
- Nanomaterial crystallite and grain size
- Nanomaterial aspect ratio / shape
- Nanomaterial specific surface area
- Nanomaterial Zeta potential
- Nanomaterial surface chemistry
- Nanomaterial dustiness
- Nanomaterial porosity
- Nanomaterial pour density
- Nanomaterial photocatalytic activity
- Nanomaterial radical formation potential
- Nanomaterial catalytic activity
- Endpoint summary
- Stability
- Biodegradation
- Bioaccumulation
- Transport and distribution
- Environmental data
- Additional information on environmental fate and behaviour
- Ecotoxicological Summary
- Aquatic toxicity
- Endpoint summary
- Short-term toxicity to fish
- Long-term toxicity to fish
- Short-term toxicity to aquatic invertebrates
- Long-term toxicity to aquatic invertebrates
- Toxicity to aquatic algae and cyanobacteria
- Toxicity to aquatic plants other than algae
- Toxicity to microorganisms
- Endocrine disrupter testing in aquatic vertebrates – in vivo
- Toxicity to other aquatic organisms
- Sediment toxicity
- Terrestrial toxicity
- Biological effects monitoring
- Biotransformation and kinetics
- Additional ecotoxological information
- Toxicological Summary
- Toxicokinetics, metabolism and distribution
- Acute Toxicity
- Irritation / corrosion
- Sensitisation
- Repeated dose toxicity
- Genetic toxicity
- Carcinogenicity
- Toxicity to reproduction
- Specific investigations
- Exposure related observations in humans
- Toxic effects on livestock and pets
- Additional toxicological data
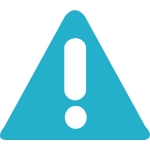
Neurotoxicity
Administrative data
Description of key information
NOAEC neurotoxicity (mouse, 6 h/day, 5 days/week, 2 a): 465 mg/m³ (CIIT, 1981)
NOAEC neurotoxicity (mouse, 22 h/day for 11 days, continuous): 207 mg/m³ (Landry et al., 1985 and expert review by Dekant and Colnot, 2013 )
Key value for chemical safety assessment
Effect on neurotoxicity: via oral route
Endpoint conclusion
- Endpoint conclusion:
- no study available
Effect on neurotoxicity: via inhalation route
Endpoint conclusion
- Endpoint conclusion:
- adverse effect observed
- Dose descriptor:
- NOAEC
- 465 mg/m³
- Study duration:
- chronic
- Species:
- mouse
- Quality of whole database:
- The available information comprises adequate and reliable (Klimisch score: 2) studies.
Effect on neurotoxicity: via dermal route
Endpoint conclusion
- Endpoint conclusion:
- no study available
Additional information
The central nervous system could be identified as one of the target organs of chloromethane. Clinical signs such as tremors and paralysis, neurofunctional impairment and histopathological findings in the brain were detected in subacute, subchronic and chronic studies.
Landry et al. (1985) exposed C57BL/6 mice continuously (22 hours/day for 11 days) to 15, 50, 100, 150, 200 and 400 (equivalent to 31, 103, 207, 310, 413 or 826 mg/m³) and intermittently (5.5 hours/day for 11 days) to 150, 400, 800, 1600 and 2400 (equivalent to 310, 826, 1652, 3304 or 4956 mg/m³) to evaluate the relationship between chloromethane exposure duration and neurotoxicity. The lowest effect level for degenerative changes in granule cells of the cerebellum for continuous exposure was 207 mg/m³ and for intermittent exposure 826 mg/m³. Greater exposure concentrations and/or exposure duration apparently resulted in a functional effect, i.e. a decrement in rotating rod performance. There were statistically significant decreases in rotating rod performance starting at 310 mg/m³ in the continuous exposure and in mice exposed intermittently for 4 days to 1625 mg/m³ and greater chloromethane concentrations. According to the authors a simple quantitative relationship between neurotoxicity and continuous versus intermittent exposure was not observed. From the results, a NOAEC of 310 mg/m³ for intermittent exposure and of 103 mg/m³ for continuous exposure can be concluded.
Kolkmann and Volk (1975) investigated chloromethane-induced neurotoxicity in guinea pigs exposed to extremely high exposure concentration for 7-70 days (41301 mg/m³ chloromethane for 10 min/day, 6 times/week).Six guinea pigs showed ataxia and paresis after 17 exposures but four did not show toxic symptoms until after 25 exposures. Numerous changes in the granular layer occurred. Focal necrosis and edema were seen after 21 days. Later on alterations in the Purkinje cells were observed and confirmed by electron microscopy.
The influence of chloromethane on the central nervous system was further investigated in a subacute study by Jiang et al. (1985). C57BL/6 mice were exposed 6 hours/day, 5 days/week for 2 weeks to 3098 mg/m³ chloromethane. Cerebellar lesions characterized by nuclear and cytoplasmatic condensation of scattered granule cells and also by watery swelling and disruption of granule cell perikarya were found. The histopathological findings were reflected in clinical observations such as deficiency in motor coordination. Few kidney abnormalities were reported, indicating that the cerebellar lesions were not secondary to kidney lesions.
In a combined chronic toxicity study (2 years, CIIT, 1981) with repeated 6-hour exposures to chloromethane severe CNS changes and brain pathology were observed in mice of the highest dose group corresponding to 2065 mg/m³. In rats no neurofunctional impairments were observed that were attributable to chloromethane exposure after two years of repeated exposure to 103, 465 or 2065 mg/m³ (for further details on the study please refer to Section Repeated Dose Toxicity).
Male cats and dogs, that were exposed 23-1/2 hours for three days following a 2 week (cats) or 4 weeks (dogs) examination time showed neurological changes, including ataxie, paralysis and tremors. The no-effect-levels were 1033 mg/m³ for cats and 413 mg/m³ for dogs (McKenna et al., 1981) (for further details on the studies please refer to Section Repeated Dose Toxicity).
Human data
In humans, the most common consequences of exposures to chloromethane have been functional changes in the central nervous system. After single or repeated exposures states described as drunkenness, although more persistent. Typical signs and symptoms of intoxication are staggering gait, weakness, double vision, headache, apathy, anorexia, nausea, vomiting, abdominal pain, diarrhea, personality changes, muscle spasms, tremors of the hands, loss of memory, paralysis and confusion have been observed. In general, the symptoms seem to develop soon after exposure. Effects of longer-term “low-level” exposure are thought to be generally mild and reversible after a recovery period (Gudmundsson, 1977; Ellenhorn and Barceloux, 1988; Sittig, 1991).
Critical assessment of data on neurotoxicity
Dekant and Colnot (2013) assessed recently for the members of the Methyl chloride REACh consortium the data on neurotoxicity and if the effects observed in rodents should be considered as relevant for classification.
Dekant and Colnot (2013) summarized that the primary target organ for chloromethane toxicity identified in humans and in rodents is the central nervous system. Moderate cerebellar degeneration with clear functional disturbances was seen in mice after continuous exposure for 11 days to concentrations of 150 ppm (equivalent to 310 mg/m³) and higher which also produced mortality (Landry et al., 1985). In life‐time studies, no cerebellar lesions or axonal dystrophy were observed in rats treated with up to 1000 ppm (equivalent to 2065 mg/m³) chloromethane (CIIT, 1981). In mice, a concentration of 1000 ppm (equivalent to 2065 mg/m³) is considered the overt effect level due to high mortality and cerebellar degenerative lesions (CIIT, 1981). Axonal swelling has been attributed to an unspecific neuronal stress response and should not be considered of primary pathophysiologic importance in the absence of manifest neurotoxicity (LoPachin et al., 2000). Furthermore, an age‐related increase of axonal swelling in the gracile tract has been reported for mice aged 8 months or over older (Bridge et al., 2009; Johnson et al., 1975), with animals aged 21‐23 months showing a high incidence of swollen axons in the nucleus gracilis, and some in the nucleus cuneatus (Johnson et al., 1975). Thus, this finding does not constitute an adverse effect and should not be considered as treatment‐related. Based on these considerations, the NOAEC for neurotoxic effects of chloromethane in mice is 100 ppm (equivalent to 207 mg/m³) when based on functional impairment in an 11‐day continuous exposure study, and 225 ppm (equivalent to 465 mg/m³) in a life‐time study with intermittent exposure (6 h/day, 5 days/week).
Furthermore, it was discussed by Dekant and Colnot (2013) whether effects on the central nervous system warrant a classification as STOT-RE. STOT‐RE is assigned on the basis of findings of “significant” or “severe” toxicity. In this context, “significant” refers to changes which clearly indicate functional disturbance or morphological changes which are toxicologically relevant (ECHA, 2012).
In two lifetime studies, the NOAEC for effects of chloromethane in rats in 250 ppm and the NOAEC for cerebellar changes in mice intermittently exposed to chloromethane (6 h/day, 5 days/week) was 225 ppm (equivalent to 465 mg/m³). Considering the guidance values given in ECHA (2012) for studies of 90‐day duration and adjusting them for the longer duration using Haber’s rule (ECHA, 2012), no classification of chloromethane for STOT‐RE is applicable.
Histopathological and adverse functional effects have been observed in mice after continuous exposure to 100 ppm (equivalent to 207 mg/m³) and higher for 22.5 hours/day for 11 days. While “slight effects” (no further information provided in Landry et al., 1985) on the cerebellum were seen in animals treated with 100 ppm, no functional impairment was noted in those animals as compared to controls when submitted to the rotating rod test. A functional impairment was noted in the same test for animals treated with 150 ppm. This concentration, however, was not tolerated as all animals were moribund by day ten and did not survive to the end of the study period. Thus, a NOAEC for neurofunctional impairment based on this study can be considered as 100 ppm, with a clear LOAEC of 150 ppm. However, this study should not be used for classification purposes due to the following reasons:
According to ECHA (2012), data for use in classification and labeling should be obtained from studies in experimental animals conforming to internationally agreed test guidelines. Generally, guidelines for repeated dose toxicity studies require exposure to the test article for 6 hours per day and 5 days/week. The use of other exposure regimens requires a clear rationale (OECD, 2009). In addition, since more weight is given to studies covering a larger part of the animal lifespan, assessment should use the results of a lifetime study when available (ECHA, 2012). Results from lifetime studies are available for chloromethane; therefore, they are preferred.
As discussed in the Section Carcinogenicity, the mode of action of chloromethane systemic toxicity in mice and rats is associated with its metabolic conjugation to glutathione. Glutathione conjugation accounts for the majority of chloromethane systemic metabolism. As shown in mice, glutathione conjugation, unusually, represents a primary toxication pathway for chloromethane: pretreatment of mice with the glutathione depleting agent buthionine sulfoximine attenuated acute chloromethane induced mortality as well as CNS, liver and kidney toxicity, indicating that parent chloromethane does not significantly contribute to toxicity (Chellman et al., 1986). Additionally, Kornbrust and Bus (1984) showed that GSH conjugation in mice is significantly higher than in rats. Although the actual toxic metabolite(s) derived from chloromethane glutathione conjugation have not been identified, methanethiol and formaldehyde have been suggested as possible putative toxic metabolites (Kornbrust and Bus, 1983; Chellman et al., 1986). Thus, the observed difference between intermittent and continuous exposure scenarios is not due to enhanced parent chloromethane toxicity, but to a constant production and accumulation of toxic metabolites in the brain. Chloromethane toxicokinetics have been studied in rats, dogs (Landry et al., 1983), and humans (Nolan et al., 1985). Overall chloromethane metabolism is proportionate to the basal metabolic rate and respiratory minute volume of these species. As demonstrated, chloromethane is metabolized far more rapidly in mice as compared to rats. Thus, humans are expected to metabolize much less chloromethane as compared to rodents on a body weight basis (Landry et al., 1985). Therefore, a direct extrapolation of observations with chloromethane in mice to humans is highly questionable.
Case studies from occupational exposure and clinical studies show that in humans concentrations greater than 200 ppm (equivalent to 413 mg/m³) are necessary for the development of chloromethane intoxication (NRC, 2012).
Continuous exposure to chloromethane is not expected for humans. Workshifts in the occupational context will not exceed 7.5‐8 hours. As most industrially‐produced chloromethane is used as a chemical intermediate (NRC, 2012), and virtually all of its commercial uses are consumptive, chloromethane is not available for release, disposal, or reuse (ATSDR, 1998). Thus, exposure of the general population by inhalation of anthropogenic chloromethane is very unlikely to occur.
In conclusion, the unusual non‐guideline experimental design with almost continuous exposure does not reflect current human exposure situations both in a worker and consumer context. While providing valuable information on the mode of action of chloromethane toxicity, the study is not suitable for classification purposes. Classification and labeling according to EU regulation 1272/2008 for chloromethane should be based on the results of the lifetime studies performed in rats and mice. Considering the guidance given in ECHA (2012), no classification with STOT‐RE regarding neurotoxicity is required for chloromethane.
References
American Conference of Governmental Industrial Hygienists (1994).Threshold Limit Values. Available at: http://www.osha.gov/dts/chemicalsampling/data/CH_252200.html [Last accessed 15/11/2012] [as cited in: Dekant and Colnot, 2013].
Bridge, K. E., Berg, N., Adalbert, R., Babetto, E., Dias, T., Spillantini, M. G., Ribchester, R. R., and Coleman, M. P. (2009). Late onset distal axonal swelling in YFP‐H transgenic mice. Neurobiol. Aging. 30, 309‐321 [as cited in: Dekant and Colnot, 2013].
Dekant W. and Colnot, T. (2013) Expert Review: Can the German OEL-value (MAK) for Chloromethane (MeCl) be considered to be equivalent to a DNEL within the REACH-framework?
Ellenhorn, M.J.and Barceloux, D.G. (1988). Medical toxicology. Diagnosis and treatment of human poisoning.: Elsevier Science Publishing Company, Inc., 982 - 983 [as cited in: US EPA, Toxicological review of methyl chloride, 2001].
European Chemicals Agency (2012). Guidance on the application of the CLP criteria. Helsinki, Finland [as cited in: Dekant and Colnot, 2013].
Gudmundsson, G. (1977). Methyl chloride poisoning 13 years later. Archives of envrionmental health 32:236 - 237 [as cited in: OECD SIDS Chloromethane, 2004].
Jiang, X.Z. et al.(1985). An ultrastructural study of lesions induced in the cerebellum of mice by inhalation exposure to methyl chloride. Neurotoxicology, 6: 93-104 [as cited in: OECD SIDS Chloromethane, 2004]
Johnson, J. E., Mehler, W. R., and Miquel, J. (1975). A fine structural study of degenerative changes in the dorsal column nuclei of aging mice. Lack of protection by vitamin E. J. Gerontol. 30, 395‐411 [as cited in: Dekant and Colnot, 2013].
Kolkman, F.W. and Volk, B. (1975) Necroses in the granular cell layer of the cerebellum due to methyl chloride intoxication in guinea pigs (author's transl.). Experimentelle Pathologie, 10(5-6):298-308 [as cited in: OECD SIDS Chloromethane, 2004]
Kornbrust, D. J. and Bus, J. S. (1984). Glutathione depletion by methyl chloride and association with lipid peroxidation in mice and rats. Toxicol. Appl. Pharmacol. 72, 388‐399 [as cited in: Dekant and Colnot, 2013].
LoPachin, R. M., Lehning, E. J., Opanashuk, L. A., and Jortner, B. S. (2000). Rate of neurotoxicant exposure determines morphologic manifestations of distal axonopathy. Toxicol. Appl. Pharmacol. 167, 75‐86 [as cited in: Dekant and Colnot, 2013].
McKenna, M.J. et al. (1981) Toxicology Research Laboratory, The Dow Chemical Company, Midland, MI 48640 [as cited in: OECD SIDS Chloromethane, 2004].
National Research Council – NRC (2012). Acute Exposure Guideline Levels for Selected AirborneChemicals, Volume 12. Washington, DC, USA [as cited in: Dekant and Colnot, 2013].
OECD (2009). Guideline for the Testing of Chemicals. Subacute Inhalation Toxicity: 28‐Day Study.Available at: http://www.oecd‐ilibrary.org/environment/oecd‐guidelines‐for‐the‐testing‐of‐chemicals‐section‐4‐health‐effects_20745788 [Last accessed 20/12/2012] [as cited in: Dekant and Colnot, 2013].
Sittig, M. (1991). Handbook of toxic and hazardous chemicals and carcinogens (3rd edition). Noyes Publication, 1086 -1088 [as cited in: US EPA, Toxicological review of methyl chloride, 2001].
Justification for selection of effect on neurotoxicity via inhalation route endpoint:
The selected study (CIIT, 1981, 2-year mouse study) is the most suitable study (for details please refer to the discussion in the Section Neurotoxicity).
Justification for classification or non-classification
No classification with STOT‐RE regarding neurotoxicity is required for chloromethane based on the above mentioned discussion (Dekant and Colnot, 2013).
However, the STOT‐RE classification is given due to Annex VI of Regulation (EC) No 1272/2008 (CLP Regulation), Table 3.1 (CLP classification) and Table 3.2 (DSD classification) (Index No. 602-001-00-7):
CLP: STOT RE 2; H373 - May cause damage to organs through prolonged or repeated exposure
DSD: Xn; R48/20 - Harmful: danger of serious damage to health by prolonged exposure through inhalation
Information on Registered Substances comes from registration dossiers which have been assigned a registration number. The assignment of a registration number does however not guarantee that the information in the dossier is correct or that the dossier is compliant with Regulation (EC) No 1907/2006 (the REACH Regulation). This information has not been reviewed or verified by the Agency or any other authority. The content is subject to change without prior notice.
Reproduction or further distribution of this information may be subject to copyright protection. Use of the information without obtaining the permission from the owner(s) of the respective information might violate the rights of the owner.
